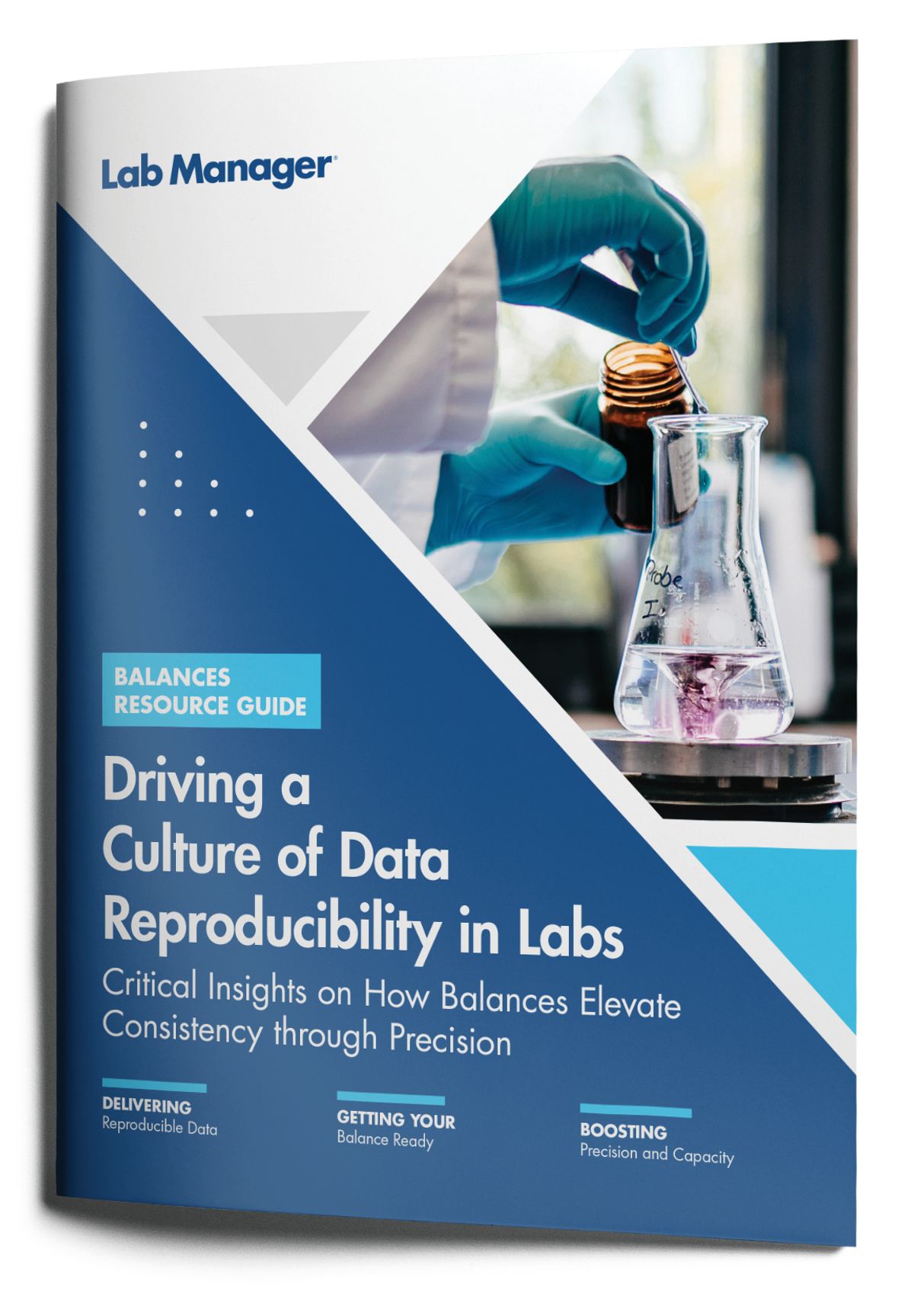
Analytical balances play a crucial role for practical applications in various industries. As high-precision instruments, balances are essential for measurements concerning the weighing of samples, and can highly influence downstream results in a laboratory’s research workflow.
Investing in high-quality balances is a must and can significantly impact research outcomes, quality, and regulatory compliance. In purchasing analytical balances, one must not only consider the lab’s specific needs but also ensure that an ideal location is available for the instrument as balances can be influenced by the slightest variations in environmental conditions.
Repeatability and reproducibility are key aspects of weighing experiments. Getting the right balance that best suits your lab’s needs will make a positive impact on the integrity of results and strengthen the accuracy of measurements.
In this eBook, you will learn about:
- How to ensure reproducible data in your laboratory
- Setting up your balance
- Establishing proper weighing practices
- Advantages, developments, and features of high-precision laboratory balances
67343_LM_Balances (A&D Weighing)_eBook_JL V3
BALANCES RESOURCE GUIDE
Driving a Culture of Data Reproducibility in Labs
Critical Insights on How Balances Elevate Consistency through Precision
DELIVERING
Reproducible Data
GETTING YOUR
Balance Ready
BOOSTING
Precision and Capacity
Table of Contents
4 Ensuring Reproducible Data in the Laboratory
11 Lab Balances: Weighing in the Wind
14 Applied Precision: A Closer Look at Laboratory Balance Technology
2 Lab Manager
17 Ultramicrobalances: The Ultimate in Precision
Introduction
Reliable and reproducible results are integral for validating experiments, product quality, and ensuring compliance with industry standards
Repeatability makes data reliable. Reproducibility validates data. Analytical balances combine these factors as sensitive instruments designed to measure mass with high precision, down to microgram or nanogram levels. Analytical balances play a significant role in various fields by providing precise measurements concerning the weighing of samples. Investing in high-qual- ity analytical balances, and those tailored to a lab’s specific needs, is paramount to achieving accurate and reliable measurements.
Ensuring repeatability and reproducibility is an integral aspect of weighing experiments. As high-precision instruments, balances can be influenced by even the slightest variations in environmental conditions. Careful adherence to standardized protocols and good weighing practices can help minimize environmental disturbances and strengthen the accuracy of measurements.
By prioritizing these factors in laboratory experiments, users can gain confidence in their work’s integrity while making a positive impact on research outcomes, knowledge dissem- ination, product quality, and regulatory compliance. Analytical balances are vital tools for fundamental research with practical applications in various industries.
This e-book serves as a guide and discusses how to ensure reproducible data in your laboratory, establish proper weighing practices, account for environmental factors that may influence your measurements, and the latest developments and features in high-precision laboratory balances.
Ensuring Reproducible Data in the Laboratory
To be credible in the scientific community and to deliver technical value to customers, laboratory managers must deliver reproducible results
by Scott Hanton, PhD
Sharing interesting observations and measurements about the world around us is at the heart of the scientific process so that others can learn and repeat the experiments. New scientific learning and theories are recognized when a consensus of scientists practicing in a specific area is reached about the ex- perimental outcomes. This consensus can only be reached if the results are reproducible. In addition, these new learnings are applied to the resources and products we use daily. Tests for quality and safety are developed to ensure appropriate and safe use. The data from the safety and quality tests need to be accurate and accepted. The experiments producing the data must be validated and reproducible to be acceptable.
In our modern world with fast, easy, and sometimes over- whelming amounts of communication, there is more visibili- ty than ever before about the need for reproducible data.
What is reproducibility?
According to the National Academy of Sciences, reproduc- ibility is obtaining consistent results using the same input data; computational steps, methods, and code; and condi- tions of analysis.1 To be reproducible, results need to follow the same procedures and control what can be reasonably controlled. Reproducibility does not mean identical results. Random elements will still impact the results, so an under-
standing of statistics and measurement uncertainty2 is still required. Reproducibility is also different from replication. Replication is an attempt to identically repeat an experiment. Figure 1 shows Zach Scott’s representation of replication and reproducibility.3
Of course, the lack of reproducibility in experiments is nonsense. “Non-reproducible single occurrences are of no significance to science.”4 To be credible in the scientific community and to deliver technical value to customers, laboratory managers must deliver reproducible results. In the end, the quality of our data is the most important part of our roles as laboratory managers.
Ensuring reproducibility
Laboratory managers have several ways to ensure their labs are producing high-quality, reproducible technical results:
High expectations
Method validation
Understanding measurement uncertainty
Effective training
Level of Similarity
Research Question
Data Model Hardware User
Replication
Reproduction
Nonsense
?Figure 1: Zach Scott’s representation of replication and reproducibility. ADAPTED FROM ZACH SCOTT'S “DATA SCIENCE’S REPRODUCIBILITY CRISIS”
Effective documentation
Good lab practices
Shared results
It is important for all laboratory managers to clearly and spe- cifically establish the expectation that the scientific outcomes delivered by the lab are of high quality, are reproducible,
and meet all the expectations of good science. Being clear about why this is important and never accepting shoddy work is a part of laboratory leadership. Laboratory managers can encounter problems when they assume their scientists understand this, or when they are too busy to be aware of the details of work in the lab.
Method validation is a key step in driving reproducible out- comes. Method validation takes advantage of all the experi- ence, talent, and knowledge of the scientists developing new methods and breaking new scientific ground to teach others how to follow the path.
Validation examination
Typical validation examines these different areas of the method:5
Accuracy- closeness of the result to the accepted value
Precision- degree of scatter from a series of measurements
Repeatability- same conditions within a short time
Intermediate Precision- reproducibility, different condi- tions over a longer time
Specificity- ability to assess the analyte in differ- ent matrices
Detection limit- lowest amount of the analyte that can be observed
Quantitation limit- lowest amount of the analyte that can be measured
Linearity- results are proportional to amounts
Range- the interval over which the method has linearity
Method validation considers both replication and repro- ducibility. Using precision measurements, the ability of the method to produce valid outcomes is determined for a
series of experiments conducted by the same person, on the same instrument(s), under the same conditions, over a short period. The same measurements are done for experiments conducted by different people, on different instruments, under similar conditions over longer periods. If the experi- ments produce valid results under these conditions, then we have significant confidence that others using the method will generate reproducible outcomes.
Measurement uncertainty provides a clear understanding of the repeatability of results. The accuracy, precision, and standard deviation of results provide a clear indication of the quality of a technical outcome. All scientific labs need to understand if the differences observed in experiments are significant. Does a set of results demonstrate that the outcomes are all the same, with some spread of uncertain- ty, or do they represent a real, although small, difference? Labs must be able to discern the difference and adequately communicate that difference to their clients or customers.
Lab managers can ensure an improved understanding of measurement uncertainty by ensuring staff receive adequate statistical training, and documenting the key measurement uncertainty terms, formulae, and calculations in an internal standard operating procedure (SOP).
Training is an important function in all labs. Despite the high quality of college education, most new employees require significant training to contribute to the lab’s work. In addition, learning organizations are constantly sharing and training across staff to improve flexibility, innovation, and agility for the lab. Training is recognized by most accredita- tion agencies as a vital part of demonstrating competency in lab work. A review of the lab training records is an important piece of most lab audits.
Ensuring effective training
Laboratory managers can ensure effective training by focus- ing on these aspects:
Choose an experienced teacher for the training
Provide training to the teachers to improve their teaching skills
Ensure the training includes actual, hands-on, practical activities as reading alone is not training
Utilize both shadowing and on-the-job (OTJ) training
Shadowing allows the student to observe as the teacher executes
OJT training allows the teacher to observe as the student executes
Effectively document important SOPs and train to them
Document, document, document. According to one of our A2LA auditors, if it wasn’t documented, it didn’t happen. Documentation is a vital step in the process. Not just to demonstrate to outsiders that effective training has occurred, but also to lay out the steps of the process, method, or experiment that needs to be completed. Most labs use SOPs to document how to properly complete the important things the lab does. These SOPs become the official how-to docu- ments that all staff are expected to understand and follow.
SOPs may be very detailed to document standard methods and known procedures that must be frequently reproduced by different people. Other SOPs may be less detailed to describe approaches to problem-solving or to provide a gen- eral outline of an approach to more complex processes, like research and development projects.
One piece of the lab documentation that is often neglected is addressing the ‘why’ questions. We are very comfortable documenting the ‘whats’ and ‘hows’ of lab work. This is the
heart of a typical lab SOP. Unfortunately, we don’t very often also document the ‘whys.’ By capturing the ‘whys,’ we help document the assumptions, and we have a better chance of capturing some of the tacit information that will allow future staff to understand and teach the details of the work.
To ensure reproducible lab outcomes, a wide variety of good laboratory practices are needed. General practices such as lab cleanliness, calibrated equipment, controlled environment, and documentation of observations and results are good practices universal to nearly all kinds of labs. Specific practices correspond to the details of the science practiced. Mike Mi- chaud is the environmental lab program manager for the City of Abilene, Texas. He suggests good practices of spike/recov- ery, triplicate experiments, and ensuring all results fall within the range of standard deviation defined for the standards.
Training and expecting scientists to follow good lab practices will help drive greater reproducibility in results.
Sharing the results of experiments with other practitioners is another way to ensure reproducible results. Round-robin test- ing is often used to compare the results on identical samples using the same method across different labs. Round-robin tests are a direct way to test reproducibility. The experiments are being completed by different people using different instru- ments in different labs having different environmental condi- tions. Round-robin tests provide data about the reproducibility of a method and provide lab managers with feedback about their lab’s ability to reproduce results.
Summary
Generating reproducible results is the key to good science. Reproducible results help verify discoveries, demonstrate competence in measurements, and enable clear communica- tion of technical outcomes to other scientists. Lab managers have several ways to ensure and improve reproducibility in their labs, including having high expectations about deliv- ering excellent science, appropriately validating methods, using proper measurement uncertainty, providing effective training, ensuring effective documentation, expecting the implementation of good lab practices, and broadly sharing technical results.
References
“Reproducibility and Repeatability in Science.” Na- tional Academies of Sciences, Engineering, and Med- icine (2019), p46, THE NATIONAL ACADEMIES
PRESS, Washington, DC
NIST. “Measurement Uncertainty.” https://www.nist.gov/ itl/sed/topic-areas/measurement-uncertainty
Zach Scott. “Data Science’s Reproducibility Cri-
sis.” https://towardsdatascience.com/data-sciences-repro- ducibility-crisis-b87792d88513
Karl Popper. “The Logic of Scientific Discovery.” Rout- ledge Classics, London, 1992
European Medicines Agency, ICH Topic Q 2 (R1) Valida- tion of Analytical Procedures, https://www.ema.europa. eu/en/documents/scientific-guideline/ich-q-2-r1-vali- dation-analytical-procedures-text-methodology-step-5_ en.pdf (1995)
Choosing the right balance and using good weighing practices, defined by an effective SOP, helps users get the best out of their weighing needs
by Ajay P. Manuel, PhD
Analytical balances feature in various scientific and in- dustrial applications. Accurate measurements surrounding research, quality control, and other processes rely heavily on adherence to good weighing practices, proper equipment calibration, sample handling, and effective monitoring of environmental controls. Choosing the right analytical bal- ance that best fits the specific needs of the user is paramount to successful research and ensures accurate, precise, and reproducible results.
Choosing your balance
Weighing needs vary based on the nature of the experiment being conducted. There are a variety of choices to pick from in the market, and their prices can vary significantly. As such, users should always consider the key features that may determine the best fit for their needs.
Weighing range
A balance must have a suitable weighing range for the sam- ples in question while avoiding overloading or excess strain. Using a balance with a proper weighing range ensures the accuracy and validity of results.
Readability
This refers to the smallest increment of weight that a balance can accurately measure. Readability is dependent on the balance’s design and construction. Higher-precision balances generally have smaller readability, enabling more precise measurements.
Application
Depending on whether the balance is to be used for sample preparation, density analysis, or pipette testing, to name
a few applications, choosing the balance that best fits your needs is crucial for your lab’s efficiency and productivity.
Verification
Always make sure that the balances you purchase have the necessary verifications and certifications to ensure regula- tory compliance. This also helps confirm that the balance is configured properly and is in working order.
Price
Look for balances that best fit within your lab’s budget while covering your needs. Overspending on balances with addi- tional features that you may not require can be wasteful. On the other hand, opting for a cheaper balance that does not meet your needs can also negatively impact lab productivity.
Before weighing your samples
It is always good for a user to ask the following questions before working on a balance:
) What is to be weighed? How large and heavy are the samples?
) What are the minimum tolerances required for the experiment?
) Does the balance have to be calibrated and/or verified?
Good weighing practices
An analytical balance is only as good as its user. Proper weighing practices, characterized by an effective SOP, are indispensable for accurate and reliable measurements.
Various environmental factors can also influence and affect the accuracy of measurements on an analytical balance. While it may be difficult to eliminate or avoid these factors, users must find means to minimize their impact. The higher the accuracy or precision required for a given weighing measurement, the more care must be taken with environmental conditions.
Environmental factors to consider
) Temperature
) Humidity and static buildup
) Drafts
) Air Pressure
) Grounding/Stability
) Vibrations
) Location
Taking into consideration all these factors, good weighing practices can go a long way in helping researchers get the best results out of their balances and include:
Place your balance on a stable and level surface
You ideally want a stable, and level surface, such as a built-in countertop or a heavy table. Even the slightest slope can significantly impact the accuracy of your readings. Balances also accompany built-in and adjustable foot screws for level- ing and stabilization.
Maintain consistent ambient temperature
Analytical balances work best when the ambient temperature does not vary too frequently. Avoid placing your balance
in areas with significant temperature variations like doors, windows, air vents, and even direct sunlight. air vents.
Check for environmental interference
Ensure the balance is placed in a location away from possible interference in the surroundings. This can include ceiling dust and magnets, to name a few. Using a discharge ionizer also helps prevent static build-up when working with dry, non-conductive samples.
Routine calibration is a must
Maintain a strict calibration schedule on a daily or weekly basis, especially whenever the balances I moved. Properly store your calibration weights in a closed container.
Use the draft shield door
All analytical balances come with a draft shield door. Use it and make sure to close it before taking your measurements. This helps to isolate the weighing pan from the environment and avoid discrepancies in your results.
Minimize user error by using the right tools
Eliminate human error from your results by always wearing gloves and using tongs or tweezers to avoid direct contact with your samples, while also making sure to avoid excessive vibrations by leaning on the table.
Follow proper cleaning practices
Spills are commonplace in a lab environment. Make sure to check and clean your balance before every measure- ment. This can be achieved using a soft brush for minor or dry spills, and non-aggressive solvents, like a mild soap, for stains.
By following these steps and guidelines, users elevate preci- sion and reproducibility in their weighing measurements and thus can deliver consistent results.
Lab Balances: Weighing in the Wind
Even a little airflow can throw off a measurement
by Mike May, PhD and Ajay P. Manuel, PhD
Accurate weighing of a sample is critical and a highly influen- tial factor in scientific ema scientific steps. The accuracy of a weighing measurement may depend on many factors includ- ing the application and the amount of sample being used. In fact, in situations when accuracy matters, even environmental variables such as airflow can easily influence a measurement.
Experts state how drafts of air and vibration not only sig- nificantly affect weighing quality but also cause a deteriora- tion in repeatability. As a result, this contributes further to measurement uncertainties in the form of weighing errors in various laboratory weighing systems. For a balance that can weigh 5-50 grams with a readability of 1 microgram, a draft can significantly influence the result.
Draft shields are not a one-stop solution, especially for analytical balances or microbalances. External air ingresses can often develop and cause turbulence within the weighing chamber when the draft shield door is open. Location also plays a crucial part in measurements. If the balance is kept in an area with significant airflow, the body of the balance or draft shield may still be affected resulting in vibrations through the balance chassis. If one plans to use a balance inside a hood, the need for blocking drafts can increase because of the higher air velocity, further emphasizing the need to handle airflow. In all these cases, experimental and measurement repeatability suffers heavily.
Meet the needs
To ensure you have the right balance to meet your laboratory’s needs, experts suggest taking the following measures:
Buy the best balance that your lab can acquire and afford.
High-end balances provide better electronic filtering capabilities, stable weight sensors, and minimize environmental effects during measurements.
Enabling the right environment for your balances. A great balance gets a scientist partway to good results, but the wrong environment can foul up the accuracy of even the best balance.
In conclusion, the level of air protection needed in weighing depends largely on the accuracy required. When the highest accuracy is needed, a balance must be in a suitable location with airflow managed as much as possible.
“For a balance that can weigh 5-50 grams with a readability of 1 microgram, a draft can significantly
influence the result. ”
Dealing with drafts
Certain steps can be taken to ensure your balance avoids drafts while providing accurate results.
Balances must be placed in the right location, ideally a heavy table with a stone top, and away from open windows and doors, and frequent personnel activity.
A shield can be implemented to keep airflow away from a sample. The shield must be small enough to not be cooled by airflow and also large enough to block.
Product Spotlight
Borealis BA series of Micro and Semi-Micro Balances from A&D make measurements and regulatory compliance as easy as ever!
Borealis BA balances combine an intuitive touchscreen user interface with advanced connectivity options and software functionality. These features ensure ease of use, effortless process integration, regulatory compliance and impressive performance all built into a space-saving design.
The BA is targeted for use in R&D and analytical labs in industries including academia, biotech, pharmaceutical, manufacturing, cosmetics, and other niche markets. Standard features include internal automatic calibration, a 5-inch color touchscreen display with 4 dedicated quick access keys, multi-pro- grammable infrared sensors, and automatic draft shield doors that are easy to remove and clean.
Other features include internal diagnostic testing such as automatic repeatability, eccentricity, and shock protection to ensure maximum precision and accuracy, all logged for traceability. The BA also offers a variety of communication options that allow flexibility to integrate with any LIMS system using the standard Bluetooth, Ethernet, USB-A, USB-B, RS232, and external device inputs.
All BA balances come with a five-year warranty.
Applied Precision: A Closer Look at Laboratory Balance Technology
A wide array of precisions, capacities, and features add up to balance options for every type of laboratory
by Marnie Willman
Laboratory balances are one of a few pieces of equipment that every lab needs regardless of its size or the application in which it is engaged. Whether for research, analytical, or pharmaceutical labs, balances are the linchpin of precise scientific measurement. The integrity and quality of exper- imental data are dependent upon the balance’s accuracy and usability. Identification and characterization of materials
are only possible if starting quantities are dependable. The selection of laboratory balances can impact the accuracy of experimental outcomes, adherence to regulatory standards, and the optimization of lab workflows.
This article reviews the specific technologies behind labora- tory balances, highlighting the importance of standards and
calibration, application-specific features, and emerging trends, including miniaturization and sensitivity enhancements.
Types of laboratory balance technology
Often grouped into categories like top loading or precision balances, analytical balances, and microbalances, laboratory balances are broadly distinguished by their underlying tech- nology, differing in configuration, sensitivity, and capacity.
Electromagnetic force restoration (EFR)
At the heart of many high-precision balances, especially analytical and microbalances, lies electromagnetic force restoration (EFR) technology. EFR balances work by gen- erating an electromagnetic field that counterbalances the mass placed on the weighing pan. This technology allows for extremely precise mass determinations-down to micro- gram levels-by measuring the current required to maintain equilibrium. EFR’s high sensitivity makes it ideal for tasks requiring the greatest accuracy, such as weighing minute quantities of chemicals for pharmaceutical compounds or bonding wires in nanofabrication.
Analytical balances often have maximum capacities of 220 grams or less and are common in chemistry and quantitative analytical labs. They’re frequently used for constituting milliliter-scale solutions, for example, cytokine and small molecule inhibitor stock solutions. These are typically placed on an anti-vibration table or platform, which reduc- es fluctuations caused by the surrounding environment, as nearby footsteps, air movement, and shifting glassware on a bench can be enough to affect the weight reading.
The drive towards miniaturization in scientific research has necessitated advances in balance sensitivity. Ultra-microbal- ances now achieve nanogram precision, a boon for fields like nanotechnology and materials science.
Load cell technology
Prevalent in top-loading balances, strain gauge load cell technology measures weight based on the deformation of a metal beam, converted into an electrical signal. This method provides durability and flexibility for weighing larger quan- tities, though with less precision than EFR. This translates to the highest capacity with the lowest measure of readability—
the smallest difference in mass that the balance can reliably indicate—usually differences of 0.01 gram (10 milligrams).
The open configuration and large pan on top loading bal- ances accommodates diverse weighing vessels. They are the workhorses of research labs and used to create a variety of solutions requiring dissolution of tens or hundreds of grams of powder. When an investigator prepares reagents for aga- rose gels and western blotting experiments, the top loading balance is a relevant piece of equipment. Although most have maximum capacities of five to 10 kilograms, there are spe- cialized high-capacity instruments that can measure weights up to 70 kilograms, convenient for taring large vessels before anything goes into them.
Regardless of technology and capacity, the reliability of balance measurements hinges on adherence to international standards such as ISO/IEC 17025 and regular calibration practices. Calibration ensures that balances perform within specified tolerances, assuring accuracy of measurements across a range of conditions.
Application-specific features
Choosing the right balance is critical to maintaining high standards of accuracy and efficiency. The specific needs of the lab and application, including the typical weight range, level of precision required, and environmental factors, should guide the selection process.
Balances with internal calibration contain mechanisms that adjust for environmental changes like temperature and pres- sure. This can improve consistency and efficiency, especially in high-throughput settings. The development of materials and coatings that reduce static interference and enhance du- rability mark further improvements in balance technology, in some instances available in custom configurations to suit a variety of environments. For example, draft shields with ionizers reduce static electricity while weighing precious powders, a critical feature for hygroscopic materials.
“...laboratory balances are broadly distinguished by their underlying technology, differing in configuration,
sensitivity, and capacity”
Wireless connectivity and network integration are increas- ingly featured in modern balances, improving data man- agement, and streamlining analytics, particularly where balances can be integrated with lab systems like LIMS. User authentication and detailed logs and reports can strengthen audit trails and facilitate regulatory compliance.
There is a plethora of options for today’s scientists, and a vast array of precisions, capacities, and features means that there is a perfect balance for every laboratory. Carefully assessing your options and working with well-versed industry profes- sionals and other balance users is the best way to ensure your lab has the ideal balance for its unique needs.
The Ultimate in Precision
If a balance’s measurement goes to seven decimal digits after zero, like 0.0000001 grams, that’s an ultramicrobalance
by Mike May, PhD and Ajay P. Manuel, PhD
An ultramicrobalance provides measurements down to 10-7 grams. That level of precision handles the smallest samples and can be used in various applications including mining, emissions testing, elemental analysis, and drug discovery. When it comes to precision, ultramicrobalances take it to the extreme. To enable researchers with this resource, these balances also require special features, guided by the applica- tions for which they will be utilized.
With drug discovery, lead-optimization processes often re- quire minute and the smallest amounts of possible new drugs to be analyzed. As a consequence, users must often try to save as much of the sample as possible since only very small amounts of samples are available in the first place for all the required analytical testing to be done. In the case of emis- sions testing, experts state that particulate matter is collected on filters, and is later weighed on microbalances. The weight of these particles is often very low with regulations requiring the use of a microbalance to procure accurate measurements.
“To get the best results from an ultramicrobalance, scientists often want a platform that provides connectivity. ”
Ultramicrobalances have also reared their head in unexpect- ed places and have been used to calibrate pipettes to volumes in the microliter range. Apart from their diverse applica- bility, ultramicrobalances have also supported the develop- ment of novel technologies as in the case where Canadian scientists used an ultramicrobalance to measure particles
in an infant’s nasal pathways. These examples demonstrate the versatility of these balances and the importance of using them correctly, in a balanced manner so to say, for the right task.
Tracking the temperature
The fine measurements enabled by ultramicrobalances depend intimately on the ambient conditions. Some of these balances are equipped with an internal ambient conditions module to track and measure humidity, pressure, and tem- perature. When ambient conditions are less than ideal, the balance would not be stable, resulting in continuously chang-
ing measurements. A good ultramicrobalance also provides for internal adjustments. Some balances are equipped with an active temperature control system allowing the tempera- ture of the weighing cell to remain stable alongside improved signal processing, leading to overall better measurement performances. The real question is how this all works out in the lab with experts identifying a microbalance’s resistance to drift as the most essential feature for its everyday use without extensive needs for continuous calibration.
Check the connections
To get the best results from an ultramicrobalance, scientists often want a platform that provides connectivity. The most common solution involves balances with module design using cables connecting particular components, although it is not the most satisfactory one. Companies have started de- veloping alternatives with features like wireless connectivity between a computer terminal and the weighing module of
a balance. Not only do these smart interfaces enable train- ing-free weighing, but they also make manual result capture obsolete.
The take-home message for ultramicrobalances is: Look for every opportunity to enhance the accuracy, repeatability, and ability to document the conditions of the measurement. Features like these must be included in a platform to ensure ongoing and precise results.
A&D Weighing is an ISO 9001-certified company based in Ann Arbor, MI with more than 40 years of experience with technology innovation in the development of precision measurement and control product inspection equipment. A&D Weighing designs and manufactures a complete line of elec- tronic balances, scales, weighing indicators, viscometers, and controllers for pharmacy, laboratory, food service, and industrial applications. A&D is a multinational company with operations in Asia, Australia, Europe, Mexico, and the United States and Canada.
In partnership with