Next generation sequencing (NGS) revolutionized genomic research, providing unprecedented speed, scalability, and efficiency in DNA sequencing. The new capabilities presented by this set of advanced technologies has accelerated scientific discovery, broadening our understanding of genetic variations, gene expression, and relationships. Each technology differs in underlying mechanics and methodologies establishing unique requirements and considerations, however, which can make navigating the field intimidating.
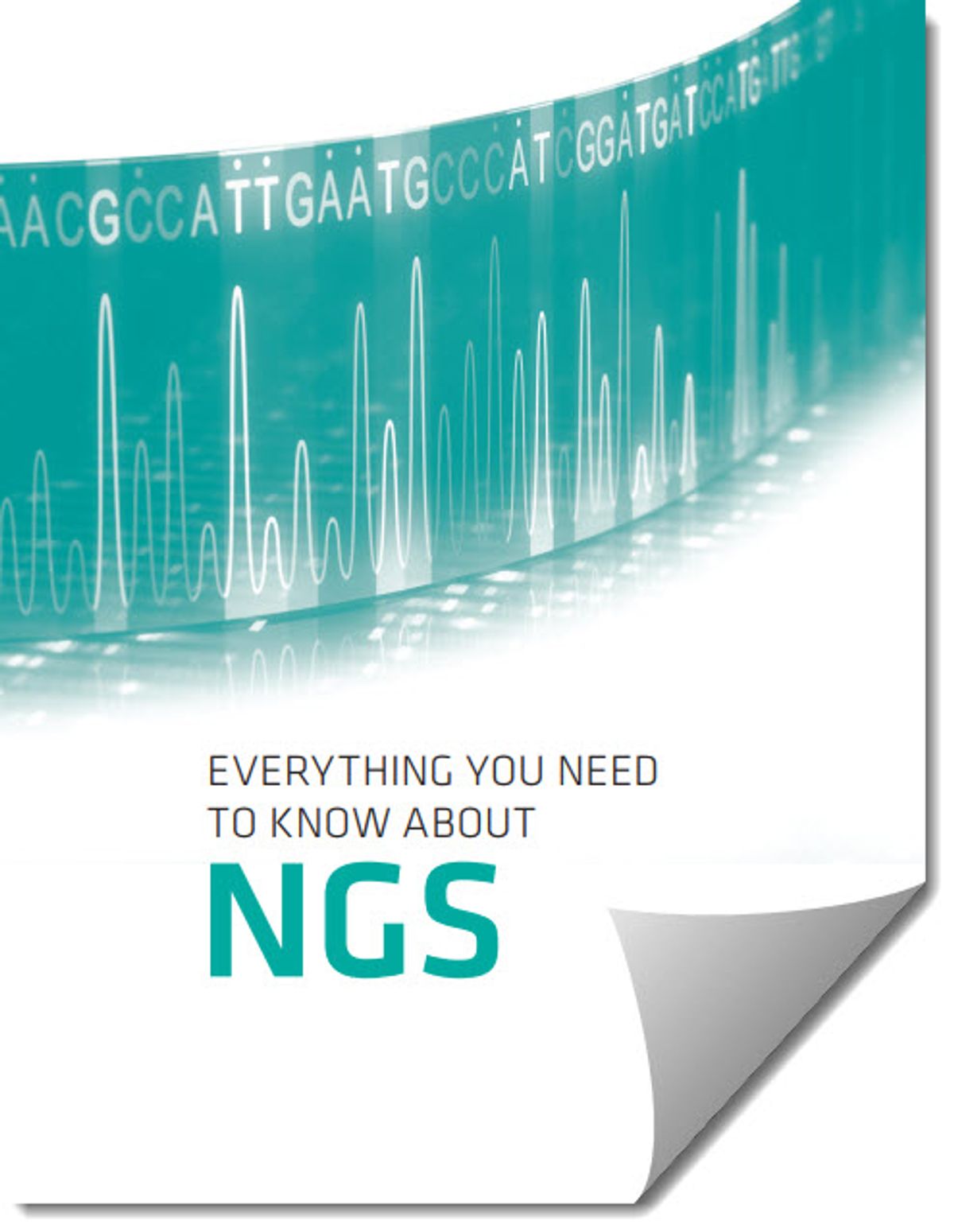
This comprehensive, illustrated eBook is designed to guide lab managers and researchers through the complexities of NGS. It offers a clear and accessible but detailed exploration of pyro-, Illumina, PacBio, and Nanopore sequencing technologies, comparing them to each other and traditional sequencing methods. It further provides several application notes with detailed workflows for improved sample and library preparation. Each chapter is crafted to enhance your knowledge and application of these techniques in your lab.
Download Everything You Need to Know about NGS now to:
- Understand the principles, advantages, and limitations of different sequencing methods through an in-depth analysis of NGS technologies.
- Learn how to integrate NGS technologies into your workflows with insights from real-world case studies and application notes.
- Discover how automated library prep and liquid handling systems can streamline your NGS processes, improving efficiency, reproducibility, and throughput.
- Leverage detailed protocols and best practices to optimize performance, including bead-based purification, automating library prep and sequencing kit workflows, high-throughput RNA barcoding, and automating size selection and DNA normalization.
1416-23-ebook-NGS-150424 (1) NGS
EVERYTHING YOU NEED TO KNOW ABOUT
FOREWORD
Next generation sequencing (NGS) has revolutionized genomics, empowering scientists with a powerful tool to sequence millions of DNA fragments at once. This transformative technology has increased the speed, scalability and throughput of sequencing, accelerating genomic research to broaden our understanding of genetic variations and gene expression.
At INTEGRA Biosciences, we recognize the pivotal role that NGS has played in shaping the future of genomics research, so we have developed a comprehensive eBook to help scientists navigate this technique. This eBook guides the reader through different sequencing methods, exploring the advantages and disadvantages of each, and provides a series of educational articles, application notes and testimonials to show how INTEGRA Biosciences' solutions can help to streamline NGS workflows.
Dr Éva Mészáros
Senior Application Specialist eva.meszaros@integra-biosciences.com
Anina Werner
Content Manager anina.werner@integra-biosciences.com
TABLE OF CONTENTS
CHAPTER 1: What you need to know about NGS
DNA sequencing methods 3
Sanger sequencing vs NGS 18
CHAPTER 2: What INTEGRA can offer 24
CHAPTER 3: Application notes
Enabling the Illumina DNA PCR-Free Library Prep kit on the
MIRO CANVAS NGS prep system 28
Automating PacBio® SMRTbell® whole genome sequencing library prep
on MIRO CANVAS 34
Automating the Oxford Nanopore Ligation Sequencing Kit on MIRO CANVAS 39
Implementing the Twist Human Core Exome Kit on MIRO CANVAS 45
PCR clean-up protocol with Agencourt AMPure XP magnetic beads 51
Beckman Coulter AMPure XP beads protocol for PCR purification 58
Automated size selection with CleanNGS, the cost-effective alternative to AMPure XP 67
Automated DNA normalization for NGS library prep 76
Affordable, high throughput bulk RNA barcoding and sequencing plate preparation
with the VIAFLO 384 84
CHAPTER 4: Customer testimonials
ASSISTing leukemia research at the Cancer Institute 92
Precise and flexible pipetting for genetic analysis and molecular biology applications 94
VIAFLO 96 and VIAFLO 384 used to provide high throughput and cost-effective
BRB sequencing 96
Technician's best friend - solving the dog epigenome to advance oncology 98
CHAPTER 5: Conclusion 100
CHAPTER 6: References 101
CHAPTER 1:
What you need to know about NGS
DNA sequencing has progressed enormously since the inception of Sanger sequencing in the 1970s, and there are now various methods on the market, each with their advantages and limitations depending on your desired application. NGS techniques were developed to
provide faster, more efficient and cost-effective sequencing methods, outperforming traditional approaches.
Before embarking on your NGS endeavor, it is important to understand the different techniques and how they can be applied to your workflow. In this chapter, we will cover the history of the different DNA sequencing methods, and delve into the difference between Sanger sequencing and NGS.
DNA sequencing methods
DNA sequencing has come a long way since its inception in the 1970s. A major milestone was marked by the Human Genome Project, which was initiated in 1990 and completed in 2003. Based primarily on the Sanger sequencing method, the project aimed to map the entire human genome, and its success has greatly advanced our understanding of human biology and genetics.1 However, as the demand for faster, more efficient and more cost-effective
sequencing methods increased, next generation sequencing (NGS) techniques that outperform Sanger sequencing were developed. This has reduced the cost of sequencing an entire human genome from $2.7 billion to just a few hundred dollars over the past couple of decades,2 making personalized medicine and genetic testing more accessible to the general public. Moreover, new research programs - such as All of Us from the National Institutes of Health (NIH), which aims to build a diverse DNA database by sequencing the genome of more than a million US citizens - have been made possible thanks to NGS approaches. In this article, we will explore the evolution of DNA sequencing methods, and explain how they work.
What is DNA sequencing?
DNA sequencing
DNA sequencing is used to determine the order of nucleotides in a DNA segment, genome, or microbiome. The steps needed to obtain the order of nucleotides depend on the sequencing method chosen, with common techniques including Sanger, Illumina, PacBio and Nanopore
sequencing.
Let's start with a general definition of DNA sequencing:
History of DNA sequencing
The history of DNA sequencing can be divided into several generations, each characterized by its own techniques and technological advancements.
First generation sequencing
The first major breakthrough in DNA sequencing was achieved in 1977 when two methods - Maxam-Gilbert and Sanger sequencing - were developed.3,4 Sanger sequencing proved to be more accurate, robust and easier to use, so quickly became the most common technology used to sequence DNA, and remained so for many years. The method is also known as chain termination sequencing, and relies on the incorporation of dideoxynucleotides into the DNA strand during replication,5 which is described in more detail below.
Next generation sequencing
Sanger sequencing is still used around the world today, but has been largely superseded by next generation or high throughput sequencing techniques, which can be divided further into second and third generation methods.
Second generation sequencing
The second generation of DNA sequencing began with the introduction of pyrosequencing by a group from the Royal Institute of Technology in Stockholm, Sweden, in the late 1990s.6 Pyrosequencing is based on the detection of pyrophosphate release during DNA synthesis.7 It was the first NGS technology to be commercialized with the introduction of a sequencer by
454 Life Sciences in 2005.6 Today the technology has been largely replaced by more advanced NGS methods that offer a higher throughput.8 One of these techniques is Illumina sequencing. It is based on the use of reversible terminator-bound dNTPs that are incorporated into the
DNA during synthesis,9 and has undergone several improvements since the launch of the first sequencing machine in 2007.10 Nowadays, Illumina sequencing is the most widely used NGS technology.11
Third generation sequencing
As we'll see below, Illumina sequencing requires a DNA amplification step, which has several disadvantages. Different research groups have therefore explored real-time, single-molecule sequencing, leading to the introduction of the two third generation sequencing techniques from Pacific Biosciences (PacBio) and Oxford Nanopore Technologies (ONT).11 PacBio sequencing monitors the incorporation of nucleotides by a polymerase enzyme in real-time,11 whereas Nanopore sequencing uses nanopores embedded in lipid membranes. When single-stranded DNA (ssDNA) molecules pass through these pores, electrical signals that vary depending on the base sequence of the molecules can be detected.12
DNA sequencing methods
As seen above, several DNA sequencing methods have been developed over the course of the last few decades. The three market leaders in DNA sequencing today are Illumina, PacBio and Nanopore. In this section, we will explain how their techniques work, after having a closer look at the Sanger and pyrosequencing methods that were developed earlier.
Sanger sequencing
Sanger sequencing was invented in 1977, and remained the main DNA sequencing method until the 21st century. It is now used less frequently, because other techniques are more affordable and efficient, but it still remains a key method for applications such as sequencing single genes or short fragments.13
Sanger sequencing
Sanger sequencing, also called the chain termination method, is a method used to determine the nucleotide sequence of DNA. The basic principle of Sanger sequencing is to synthesize a new strand of DNA complementary to the template strand, while incorporating modified, labeled nucleotides that can terminate the synthesis process. Sanger sequencing can be broken down into three steps: chain termination thermal cycling, size separation and determination of the
DNA sequence.
What is Sanger sequencing and how does it work?
The first step, the chain termination thermal cycling reaction, is very similar to a standard PCR protocol. However, during master mix preparation, you not only need to add dNTPs to your reaction mixture, but also a small concentration of fluorescently labeled ddNTPs. There are two differences between these two components:
dNTPs have a hydroxyl group (OH) that allows the next nucleotide to be added to it, while ddNTPs do not.
ddNTPs are labelled with different colored fluorescent dyes for each base.12,14
dNTPs ddNTPs
Primer
dNTPs
Sample DNA
ddNTPs
PCR amplification
Chain-termination PCR products
During the extension phase of the chain termination thermal cycling reaction, the DNA polymerase creates complementary copies of the sample DNA, by adding dNTPs until it terminates synthesis by adding a ddNTP. At this point, no additional nucleotides can be added, because of the lack of a hydroxyl group. The incorporation of ddNTPs is random but, as a number of thermal cycles are performed - leading to the creation of billions of templates - it's almost guaranteed that a ddNTP has been added to all the different nucleotide positions at least once.12,14
Once the chain termination thermal cycling reaction is finished, the differently sized DNA fragments are run through a capillary containing a gel (polymer). The smaller the DNA fragments, the faster they travel through the pores in the web of the polymer, allowing you to separate them by size. At the end of the capillary, a laser beam and a detector register the color of the dye attached to the ddNTPs in a chromatogram, allowing you to build the sequence of the original DNA sample.14
Chromatogram
A G G T A C
Today, the entire process of size separation and analysis is automated, and software reads the chromatogram for you. However, when the method was developed in the 1970s, the protocol was slightly different. As the four ddNTPs were all labeled with the same radioactive dye, scientists had to perform four chain termination PCR reactions with every sample, adding only one of the four ddNTPs to each tube. The DNA segments of the four reactions were then run
in four separate lanes of a gel, and the resulting bands were visualized. This allowed you to manually determine the sequence of the original DNA sample nucleotide by nucleotide.15 For example, in the example image below, the DNA segment travelling furthest produced a band in the lane 'C' which means that the first nucleotide would be cytosine, and so on.
Gel electrophoresis
A G G T
A
C
A G C T
Pyrosequencing
Pyrosequencing was developed about 20 years after Sanger sequencing, and quickly became a standard DNA sequencing method before being replaced by more advanced high throughput techniques. Although the technique is no longer commonly used in laboratories today, we will quickly explain its principle.
What is pyrosequencing and how does it work?
The first step of a pyrosequencing protocol is the PCR amplification of the DNA sequence of interest. One of the primers used for the reaction needs to be biotinylated to allow you to subsequently capture all the forward or reverse strands using streptavidin-coated magnetic
beads. These biotinylated ssDNA sequences are then hybridized with sequencing primers.16,17
The next step of a pyrosequencing protocol is the incubation of these ssDNA sequences and sequencing primers with several enzymes and substrates.17 The first of the four dNTPs is then added to the mixture. If the DNA polymerase incorporates one or more of these dNTPs because they match the template strand, pyrophosphate (PPi) is released, resulting in an enzymatic reaction that produces light. This signal can be detected by a charge coupled device (CCD) camera, with the height of the peak proportional to the number of nucleotides incorporated.17
The next step is the addition of an enzyme called apyrase, which degrades unincorporated dNTPs. As soon as this happens, the next dNTP can be added. Once you have sequentially added every dNTP several times, you can determine the nucleotide sequence from the signal peaks.17
Nucleotide sequence
A GG - T A - C -
A G C T A G C T
Nucleotide added
Illumina sequencing
A major disadvantage of the pyrosequencing method is that only one dNTP type can be added at a time. This problem was solved by Illumina sequencing.
Illumina sequencing
Illumina sequencing is a synthesis method used to sequence millions of DNA fragments in parallel. For a standard Illumina protocol, a library is prepared by fragmenting, purifying and amplifying the DNA sample. The sequence of each of these fragments is then determined
simultaneously, and computationally aligned against a 'normal reference' genome.
What is Illumina sequencing and how does it work?
That's Illumina sequencing in a nutshell. Let's look at it in more detail to gain a more detailed understanding of this important sequencing method.
Standard Illumina sequencing can be split into the following steps:
DNA fragmentation
Addition of primer binding sites and capture sequences
DNA denaturation
DNA immobilization
DNA amplification
DNA sequencing
The first three steps are commonly referred to as library preparation, and convert the DNA sample into a library of fragments that can be handled by an Illumina sequencing machine. Low throughput labs can either perform these steps manually or use a small automated benchtop device for library preparation, while high throughput labs generally use large liquid handling robots.
Step 1: You first need to fragment your DNA sample into small pieces using either physical or enzymatic fragmentation methods.18
Step 2: The DNA sequences then need to be elongated with adapter sequences on both ends. The inner adapter sequences are primer binding sites that are used for the sequencing reaction later on, and the outer adapter sequences are capture sequences required for the immobilization step.12 As the adapter sequences are added using enzymatic reactions - where reagents, adapter sequences and enzymes are added in excess - subsequent purification steps are needed to separate the DNA fragments from unused reaction components.
Primer binding sites
Capture sequence
Sample DNA
Capture sequence
Step 3: Once you have added the adapter sequences to your sample, denature the sequences to separate the two strands from one another.12
Step 4: Your sample can then be added to the Illumina flow cell. The flow cell is pre-spotted with short DNA sequences (oligonucleotides or oligos) that are complementary to the capture sequences attached to your DNA sample, and consequently bind your sample sequences.
Once your sample sequences are attached to the flow cell, you can perform a single PCR cycle to copy them (step 1 in the image below). After this step, denature the sequences and wash out the sample DNA strands to be left with newly synthesized strands that are immobilized to the flow cell (step 2 in the image below).12
1) 2)
Step 5: These immobilized sequences need to be amplified before DNA sequencing, a step that is also referred to as cluster generation. To do this, perform several PCR cycles. The capture sequences on the flow cell are used as primers. This means that the immobilized sequences form a bridge to anneal to them during the annealing phase (step 1 in the image below). During the extension phase, the bridge is copied (step 2 in the image below) and, during the denaturation phase, the two sequences separate from one another (step 3 in the image below). Once you have about a thousand copies of each sequence in a cluster, cleave off one of the primers to ensure that you are left with only the forward or reverse strands (step 4 in the image below).12
3)
4)
1) 2)
Step 6: The sequencing process can now begin. Add a sequencing primer complementary to the primer binding site adapter on to the flow cell (step 1 in the image below). The sequencing process that then follows is similar to Sanger sequencing. A polymerase enzyme copies the template strand and adds in the first base. As this base lacks a hydroxyl group, the reaction stops, giving you time to take four images - one for green, one for black, one for blue and
1)
2)
3)
4)
one for red - to determine which base was added (step 2 in the image below). In contrast to Sanger sequencing, however, the missing hydroxyl group can subsequently be regenerated with chemicals. These chemicals also remove the fluorescent dye from the base that was incorporated (step 3 in the image below). As soon as the hydroxyl group has been regenerated, the polymerase enzyme adds the next base (step 4 in the image below), and the next imaging and hydroxyl group regeneration steps can take place.12
Library preparation
Sample 1
Sample 2
Pooling and amplification
Differentiate reads
Sample 1 Sample 2
Please note that you can sequence several samples in parallel using a single flow cell. However, if you want to perform a multiplexed assay, index sequences need to be added in between the primer binding sites and capture sequences during library preparation. These index sequences can be used as 'barcodes', so that each fragment can be identified and sorted after amplification and sequencing.19
You need to normalize the samples before pooling them, to ensure that they all have the same DNA concentration.
PacBio sequencing
Illumina sequencing includes a template amplification step that has several downsides, such as copying errors, and added time and complexity. PacBio sequencing, which allows for single- molecule real-time sequencing, has been developed to solve these issues.11
What is PacBio sequencing and how does it work?
PacBio sequencing, also called SMRT (single-molecule real-time) sequencing,20 monitors the incorporation of nucleotides by a polymerase enzyme in real time.11
Ligating adapter
Template DNA
Primer
Polymerase
Library preparation for PacBio sequencing is quick and easy to perform, as a PCR reaction is not needed. All you need to do is ligate adapter sequences to the double stranded template DNA fragments that you want to sequence. This allows you to get a SMRTbell library with circular DNA templates. Primers and polymerase enzymes that bind to the adapter sequences can then be added, and the polymerase enzymes can be immobilized to tiny wells called zero- mode waveguides (ZMWs) using magnetic beads, for example.20,21
Millions of these ZMWs form a SMRT cell that needs to be inserted into a PacBio sequencing system for analysis.20 Within the instrument, a camera monitors the incorporation of fluorescently labeled bases by the DNA polymerase. As each base is labelled with a different dye, the wavelength of the signal that is emitted when the nucleotide is incorporated allows you to determine the sequence of your template DNA. However, because the polymerase enzyme sometimes incorporates a wrong base, you should allow it to read the circular DNA sequence several times, so that you can compare the different reads with each other and eliminate errors.12
Nanopore sequencing
A second DNA sequencing method allowing for single-molecule real-time sequencing is the Nanopore technology. What is striking when you look at a Nanopore sequencers is their size. The smallest device is about as small as a remote control, and can therefore be taken into the field.12 If you are now wondering how such a small machine can sequence DNA, you are in the right place.
What is Nanopore sequencing and how does it work?
In contrast to all the other sequencing methods discussed so far, Nanopore sequencing doesn't use polymerase enzymes and labeled bases but - as the name suggests - nanopores and electric currents. But let's first have a look at library preparation.
Before pipetting your sample into the sequencer, you need to add adapter sequences that will allow the binding of a motor protein to your DNA fragments.22 This DNA library can then be added to the sequencing device that contains a flow cell with an array of tiny holes - nanopores - embedded in an electro-resistant lipid membrane. As a constant voltage is applied to the flow cell, an electric current travels through the pores, threading negatively charged DNA through them.12,23 The motor protein is needed to unwind the DNA before it passes the pore, and also controls the speed, ensuring that the DNA molecule travels through the pore step-by-step
or base-by-base.23 As the DNA disrupts the electric current in a specific manner depending on its base sequence, this will allow you to determine the nucleotide sequence of your DNA,
depending on the current that's read by detectors.12 Please note that Nanopore sequencing is, so far, the only technology that can also be used to directly sequence RNA.
Conclusion
A lot has happened in the field of DNA sequencing since the invention of Sanger sequencing in 1977 and the launch of the Human Genome Project in 1990. While it took us 13 years and
$2.7 billion to sequence the first human genome, this process could be completed within 48 hours today, and for only $600.2,12 This is only possible thanks to numerous scientific advances, and we hope that this article was able to give you a good overview of the different DNA sequencing methods over time, as well as their mechanisms.
Sanger sequencing vs NGS
From the pioneering work of Sanger sequencing to the cutting-edge advancements in next generation sequencing (NGS) technologies, we have come a long way in our ability to decipher the genetic code. Today, scientists working in the field of genomics have an array of sequencing methods at their disposal, each with unique strengths and limitations. To help you choose the right one for your application, we will compare Sanger sequencing with NGS techniques in this article, before discussing the differences between Illumina, PacBio and Nanopore sequencing. Please note that we won't be explaining how the various methods work; for more on that, please refer to our previous article 'DNA sequencing methods'.
What is Sanger sequencing used for?
Sanger sequencing was invented in 1977 and, despite the development of several NGS methods - such as Illumina, PacBio and Nanopore sequencing - since that time, it is still being used in laboratories today. For example, clinical laboratories often rely on Sanger sequencing to validate gene variants that have been identified by NGS using Illumina platforms, primarily due to the method's unparalleled accuracy of 99.99 percent.1 However, there is a growing debate about the need to double-check variants, and so throughput is increasingly becoming the key differentiator between the methods.2,3 Because Sanger sequencing can only sequence a single fragment at a time, Illumina's NGS technology - which can sequence millions of
DNA molecules simultaneously - is preferred for high throughput applications.3 On the other hand, Sanger sequencing remains popular for sequencing a short region in a small number of samples, because it can accurately read up to 1,000 base pairs without requiring complex data analysis.4,5
The simpler data analysis of Sanger sequencing, in combination with longer reads - Illumina sequencing typically only generates reads up to 300 bp - is especially useful for sequences with repeats.3 These repeats can be determined more easily with Sanger
sequencing, because the Illumina technique requires many short overlapping sequences to be linked together, a task that can be difficult, especially when the repeat sequence is longer than the read sequence.3,6
Another advantage of Sanger sequencers is that they are usually more affordable than Illumina instruments.7 On the other hand, the cost of sequencing 1,000 bases is
considerably higher on a Sanger sequencer than on an Illumina instrument,8 so throughput will ultimately determine what makes financial sense for long term investment.
In summary, the main benefits and limitations of Sanger sequencing compared to Illumina sequencing are:
Benefits of Sanger vs Illumina
Simple data analysis
Can better deal with repeat sequences
Sanger sequencers are more affordable than Illumina instruments
Limitations of Sanger vs Illumina
Low throughput
The cost of sequencing 1,000 bases is orders of magnitude higher than on Illumina instruments
We've just seen that Sanger sequencing is preferred over Illumina sequencing when dealing with repeats. However, the two long-read NGS methods - PacBio and Nanopore sequencing - are even better suited for this,9 as their read lengths outperform Sanger sequencing.10
An advantage that all three NGS methods have over Sanger sequencing is higher sensitivity. With Sanger sequencing, one chromatogram is generated for all the molecules being sequenced.7 This makes analysis easy, but variants are only detected when they occur in about 15 to 20 percent of the sequences.3 NGS methods have a much lower detection limit, because they analyze the nucleotide sequence of each molecule individually.7 With Illumina sequencing, for example, the detection limit is 1 percent.3
Illumina vs PacBio sequencing
Shifting our attention to NGS methods, we will now examine Illumina and PacBio sequencing side by side. As seen above, Illumina is a short-read sequencing technology offering read lengths ranging between 50 and 300 bp.11,12 On the other hand, PacBio excels in long-read sequencing, possessing the impressive capability of generating read lengths of 15,000 to 20,000 bp.13 It's estimated that the short-read sequencing market size is still more than 11 times bigger than the long-read sequencing market,14,15 meaning that Illumina sequencing is undoubtedly the prevalent method. However, the longer read length of PacBio sequencing can be a huge advantage when dealing with genomes that lack a high quality reference genome, possess numerous repeat sequences, or contain rare variants. Illumina's approach of assembling short reads can be labor intensive in these cases, whereas PacBio's ability to generate long reads enables easier genome assembly and increases accuracy.12
Short read sequencing Long read sequencing
Another advantage of PacBio is its real-time sequencing ability. By monitoring the time lapse between base incorporations, PacBio can detect base modifications such as methylation.16,17,18
When it comes to throughput, however, PacBio falls short. Its Revio system can deliver up to 360 Gb of reads per run,13 whereas Illumina's NovaSeq X machines can produce up to
16 Tb of output per run.19 The cost per million bases therefore tends to be higher with PacBio sequencing than with Illumina technology.
Please note that Illumina now also offers long-read sequencing20 whereas PacBio - just like Oxford Nanopore - recently entered the short-read sequencing market.21,22 However, as Illumina technology cannot compete with the read length of PacBio and Nanopore sequencing yet23
- and as PacBio's and Oxford Nanopore's short-read sequencing platforms are new in the market22,24 - we will not go into more detail about these three technologies in this article.
PacBio vs Nanopore sequencing
Let's now explore how PacBio stands against Nanopore sequencing. Both PacBio and Nanopore technologies are widely used for de novo assembly, transcriptome analysis (including gene isoform identification), and metagenomics analysis, primarily due to their ability to generate long read lengths. Moreover, the long read length is beneficial when assembling genomes that include large stretches of repetitive regions.9
Despite their utility for these applications, both technologies have relatively high error rates. While PacBio can reduce errors through circular consensus reads,9,16 Nanopore sequencing compensates for its higher error rate by combining duplex reads from a template and the complement strand of a single DNA molecule to produce a consensus sequence with fewer errors.25 However, the type of errors differs between the techniques, as PacBio errors are random, while Nanopore errors can mainly be found in homopolymer regions.26 This makes it easier to eliminate PacBio errors, and so this method achieves more accurate results.
Nanopore may be inferior to PacBio when it comes to accuracy, but it also offers some advantages. It can directly sequence RNA, clearly stands out with the longest read length ever created - an astonishing 2.3 million bases27 - and lacks PCR amplification bias, as no
amplification step is necessary.26 As the amplification efficiency of fragments with high GC or AT contents is usually lower in PCR, they are less abundant after a few thermal cycles, and PacBio sequencing may consequently underestimate their quantity - a risk that can be eliminated with Nanopore sequencing.
Furthermore, Oxford Nanopore offers portable devices at a low price16 and uses a simple library preparation process, making this technology an ideal choice for research in remote regions or mobile diagnostic labs. For example, Nanopore sequencers have been used during an Ebola outbreak in West Africa, in Antarctica, and even aboard the International Space Station.9,28
Nanopore vs Illumina sequencing
In the final section of this article, we compare Nanopore against Illumina sequencing. Nanopore can directly sequence RNA, and shines with its long-read capability, making it useful for
de novo assembly, transcriptase analysis and metagenomics. However, Illumina offers a higher throughput, making it the go-to choice for large-scale applications.
Although, as stated earlier, Nanopore sequencing doesn't require PCR amplification, eliminating amplification bias and simplifying sequencing protocols. The simple workflow, combined with the portability of some Nanopore sequencers, make it the ideal sequencing method to be used in the field or in mobile, temporary labs.
Conclusion
The choice between Illumina, PacBio and Nanopore sequencing isn't about declaring a single winner. Instead, it's about understanding that each technology has a unique set of strengths tailored for specific applications. As lab professionals, it's your role to match these capabilities to your research needs, ensuring that you are equipped with the best tool to unravel the mysteries of the genetic world.
CHAPTER 2:
What INTEGRA can offer
Sample and library preparation for NGS involves a number of complex, multi-step processes that are labor-intensive, tedious and error-prone when performed manually. Fortunately, automating these steps and working with 96 and 384 channel pipettes can help to greatly enhance the throughput and efficiency of NGS workflows, while improving the reproducibility of results. Here, we will explain how our diverse range of automated library prep and liquid handling instruments offers solutions for everyone to seamlessly improve their workflows, regardless of throughput or automation requirements.
MIRO CANVAS NGS prep system
The MIRO CANVAS NGS prep system can overcome tedious sample preparation steps by providing full walkaway automation of NGS library prep and target enrichment hybridization protocols. This is the ultimate solution to streamline costs and optimize your time, requiring only 15 minutes of hands-on work per run and reducing reagent consumption by up to 75 %.
Learn more about
MIRO CANVAS
MINI 96, VIAFLO 96 and VIAFLO 384
Manually pipetting large quantities of samples and reagents can complicate NGS workflows and increase the risk of human error. INTEGRA offers a number of multichannel electronic pipettes that can transfer multiple samples in parallel to streamline operations. The MINI 96 and VIAFLO 96 multichannel electronic pipettes transfer 96 samples, while the VIAFLO 384 can work with either 96 or 384 pipetting heads, creating the ideal solution for high throughput labs looking to streamline their nucleic acid extraction, PCR set-up or magnetic bead purification workflows.
Learn more about
MINI 96, VIAFLO 96
and VIAFLO 384
ASSIST PLUS
The ASSIST PLUS pipetting robot can help you to elevate your NGS workflows even further, by automating your pipetting protocols. The pipetting robot automates liquid handling with VIAFLO multichannel electronic pipettes and VOYAGER electronic adjustable tip spacing pipette, as well as the D-ONE single channel pipetting module. This versatile platform can be used to improve the pipetting efficiency of different stages in an NGS workflow, including nucleic acid extraction, PCR set-up, magnetic bead purification, and library normalization and pooling.
Learn more about
ASSIST PLUS
GRIPTIPS® pipette tips and reagent reservoirs
GRIPTIPS pipette tips and reagent reservoirs from INTEGRA Biosciences provide the ideal accompaniment to your INTEGRA pipetting platforms. GRIPTIPS pipette tips are free from RNAase, DNAase and PCR inhibitors, and are designed to fit perfectly on the pipettes and pipetting heads, reducing the risk of contamination or reagent wastage from tips that leak or fall off. INTEGRA's range of multichannel, divided, 12 well and automation-friendly reagent reservoirs are available in a variety of volumes, and feature visible markings for accurate measurements and to prevent overpouring. Moreover, many of the reservoir options are available with a SureFlo™ anti-sealing array, reducing dead volumes to save reagents.
Learn more about GRIPTIPS
Learn more about
REAGENT RESERVOIRS
CHAPTER 3:
Application notes
At INTEGRA, we are always keen to share our knowledge and experience to help drive scientific advancements. In this chapter, we've supplied a selection of application notes that explain the best practices for our NGS library prep and pipetting solutions, and explain how they can help to streamline your workflows.
Enabling the Illumina DNA PCR-Free Library Prep kit on the MIRO CANVAS® NGS prep system
Automate your NGS library preparation
There is an increasing demand for next generation sequencing (NGS) library preparation protocols that do not include PCR to avoid the introduction of PCR bias into the pool of DNA for sequencing.1
The Illumina® DNA PCR-Free Prep kit follows a PCR-free workflow and is being increasingly used in sensitive applications such as whole genome sequencing (WGS) because it is both flexible and easy to automate.2
Its on-bead tagmentation step is especially important for reducing library preparation time and sample input requirements. These features are of great interest for clinical applications such as tumor evaluations and newborn diagnostics, and are also important for research uses.
MIRO CANVAS is a digital microfluidics (DMF) platform that allows custom low throughput workflow automation for complex protocols such as NGS library preparation.
The system is compatible with a wide range of reagents.3 This application note describes the results that can be expected when using the Illumina DNA PCR-Free
Prep kit in a protocol developed for MIRO CANVAS.
The resulting research use only libraries can then be sequenced using Illumina platforms.
Key benefits
Library preparation with the Illumina DNA PCR-Free Prep kit is fully automated on MIRO CANVAS.
This protocol has been demonstrated on MIRO CANVAS using 50-500 ng DNA
inputs.
Sequencing metrics of libraries prepared on MIRO CANVAS using this protocol are comparable to those of manually prepared libraries.
Automation on MIRO CANVAS reduces the amount of hands-on time required for library preparation by >60 % when using this protocol.
Overview: How to enable the Illumina DNA PCR-Free Library Prep kit on MIRO CANVAS
Experimental set-up
The Illumina DNA PCR free library prep protocol was designed for fully automated use on MIRO CANVAS and has been tested using high quality DNA inputs within the 50-500 ng range. DNA should be quantified using a Broad-Range Qubit quantification kit (or similar) before beginning the protocol. Libraries are quantified using ssDNA Qubit Quantification kit or qPCR. Tagmentation, post-tagmentation cleanup, ligation and library cleanup steps are all automated on MIRO CANVAS (Figure 1). Downstream normalization and pooling require hands-on time.
Hands-on
15 MIN
AUTOMATION
2 HR
Tagmentation • Post-tagmentation clean-up • Ligation • Library clean-up
Figure 1: Experimental set-up. The MIRO CANVAS automates all the steps following reaction set-up, including tagmentation, post-tagmentation clean-up, ligation and library clean-up.
Results
Automating the experimental workflow on MIRO CANVAS produces library yields and insert sizes that result in quality sequencing metrics. The Illumina DNA PCR-Free Prep protocol for standard input has been modified and tested using 50-500 ng NA12878 gDNA* on
MIRO CANVAS. In this modified version (Table 1), combining the standard input protocol volumes of DNA input and bead-linked transposomes PCR-free (BLT-PF) with low input single-sided bead purification (1.8x ratio) was determined to be optimal for obtaining libraries
of an ideal size and with sufficient quantity for sequencing (Table 2). Libraries prepared with as low as 50 ng of input gDNA were sequenced on a NovaSeq S4 PE150. The 1.8x ratio resulted in the kit's expected insert size of ~450 bp for >300 ng input. BLT-PF and DNA input volumes will need to be further adjusted for 50 ng input to achieve the expected insert size in both manual preparation and the automated workflow on MIRO CANVAS.
*NA12878 gDNA samples were obtained from the NIGMS Human Genetic Cell Repository at the Coriell Institute for Medical Research.
Table 1: Conditions for sample purification bead addition and insert size selection across different inputs of DNA tested.
DNA VOLUME INPUT
BLT VOLUME INPUT
VOLUME INTO FIRST CLEAN-UP
FOLD FIRST CLEAN-UP
VOLUME FIRST IPB
TOTAL VOLUME INTO SECOND CLEAN-UP
FOLD SECOND CLEAN-UP
VOLUME SECOND IPB
Standard input
25 μl
15 μl
45 μl
0.8
36 μl
76 μl
1.8
42 μl
Low input
30 μl
10 μl
45 μl
1.8
81 μl
N/A
N/A
N/A
Modified version
25 μl
15 μl
45 μl
1.8
81 μl
N/A
N/A
N/A
Table 2: Library insert sizes and yields generated from different inputs of unsheared NA12878 DNA.
MANUAL
MIRO CANVAS
TOTAL WORKFLOW TIME
1 HR 45 MIN
1 HR 55 MIN
DNA input amount (ng)
500
300
50
500
300
50
Mean yield (nM)
34.4
26.7
5.3
26.2
17.8
5.3
Median insert size (bp)
496
475
364
454
447
285
50 ng
300 ng
500 ng
Input Amount
100
98
96
94
92
90
88
86
% Q30 bases Autosome callability
% Mapped reads
% Bases covered at 20X
% Coverage uniformity
% Unique reads
84
Manual MIRO CANVAS Manual MIRO CANVAS Manual MIRO CANVAS
Figure 2: Sequencing metrics performance across a range of input DNA.
Illumina DNA PCR-free libraries prepared from a range of DNA inputs using either manual or MIRO CANVAS preparation methods demonstrate comparable % Q30 score, autosome callability, % mapped reads, % of bases covered at 20X, coverage uniformity and % unique reads.
The resulting sequencing metrics values are comparable between manually prepared libraries and those generated using the automated workflow on MIRO CANVAS (Figure 2). For DNA input amounts >300 ng, MIRO CANVAS libraries match or exceed the sequencing metrics for manual libraries including base call accuracy, passing genotype calls in autosomal chromosomes, reads that confidently map to the reference genome, the % of bases covered at 20X, uniformity of coverage and duplication rates. QC metrics used in applications aimed at variant detection were additionally examined after subsampling to 40X sequencing coverage (Table 3). MIRO CANVAS libraries presented equal or better F1 scores for both SNVs and INDELs, as well as % bases covered at 20X across all input ranges of tested DNA input.
Table 3: The performance of QC metrics relevant for variant calling across a range of input DNA. All samples were subsampled to 40X sequencing coverage.
SAMPLE ID
SEQUENCED BASES (GB)
AVERAGE AUTOSOMAL COVERAGE
% BASES COVERED AT 20x
AVERAGE MITOCHONDRIAL COVERAGE
TOTAL NUMBER SNVs
SNVs
F1 SCORE
TOTAL NUMBER INDELs
INDELs F1 SCORE
Manual 500 ng
127
35.29
94.75
7527.37
4,052,452
99.90 %
25,511
99.55 %
MIRO CANVAS
500 ng
126
35.54
94.86
6023.38
4,055,818
99.90 %
25,565
99.59 %
Manual 300 ng
127
35.31
94.87
6667.97
4,051,961
99.90 %
25,473
99.57 %
MIRO CANVAS
300 ng
127
36.21
95.03
6947.82
4,054,773
99.91 %
25,563
99.59 %
Manual 50 ng
122
34.34
94.18
9902.86
4,053,721
99.88 %
25,772
99.50 %
MIRO CANVAS
50 ng
123
34.68
94.56
7956.68
4,054,906
99.89 %
25,643
99.54 %
MIRO CANVAS walk away automation reduces hands-on time
The total time required for library preparation with the Illumina DNA PCR-Free Prep kit is
25 minutes greater when automating on MIRO CANVAS, but the hands-on time is considerably less than for manual preparation workflows (Table 1). Automation with MIRO CANVAS reduces hands-on time to zero for the tagmentation, post-tagmentation cleanup, ligation, and library cleanup steps.
40 min • 27 steps
LIBRARY CLEAN-UP
CLEAN-UP CONTINUED
CLEAN-UP CONTINUED
Researcher using MIRO CANVAS
Researcher manually preparing samples
Vortex Incubate at RT Incubate until purification for 5 min supernatant is
beads clear (5 min)
Add purification Place on magnet Remove and beads to sample discard
supernatant
Hands-on
15 MIN
Prepare
reagents Hands-on
10 MIN
Pipette to mix beads
Incubate until supernatant is clear (5 min)
Air dry 2 min
Post tagmentation setup
Remove and Remove from discard magnet
supernatant
Hands-on
35 MIN
Add resuspension buffer
Add ethanol
MIRO CANVAS
automation run in progress
Pipette to Wait 30 seconds resuspend beads
Ligation
Hands-on
20 MIN
Remove and discard supernatant
Incubate at RT for 2 min
Add ethanol
Seal tube and centrifuge at 280 x g
Library cleanup
Wait 30 seconds Place on magnet
Hands-on
40 MIN
115 MIN
Remove and Incubate until discard supernatant is
supernatant clear (2 min)
Collect library
Seal tube and Prepare and label
centrifuge at new tube 280 x g
Transfer
Place on magnet supernatant to
new tube
Figure 3: Average time requirements when manually preparing libraries or automating library preparation on MIRO CANVAS with the Illumina DNA PCR-Free Prep kit.
Conclusion
MIRO CANVAS is an advanced DMF platform that can be used to automate library preparation with the Illumina DNA PCR-Free Prep kit. When using the Illumina DNA PCR free library prep protocol for MIRO CANVAS, the protocol is fully automated from the tagmentation incubation step to elution, and can be used with DNA inputs ranging from 50-500 ng.
MIRO CANVAS and manual library preparation yield comparable results, but the true walk-away automation and minimal hands-on time provided by MIRO CANVAS makes it a valuable addition to any laboratory.
For more information and a list of materials used, please refer to our website.
Automating PacBio® SMRTbell® whole genome sequencing library prep on MIRO CANVAS®
Convenient sample preparation for long read sequencing
Long read sequencing plays an important role in generating contiguous, high quality genomes for haplotype phasing, structural variant detection, and de novo assemblies.¹ Additionally, long read libraries that are prepared without PCR amplification avoid a common source of base composition bias in sequencing data.² Many long read library prep workflows have traditionally used a gel-based size selection to efficiently remove small molecules from the library.
However, this type of size selection generally requires large DNA inputs and is not automatable. The PacBio SMRTbell Prep Kit 3.0 combines the advantages of PCR-free long read sequencing with a streamlined protocol and fast bead-based size selection for an easily automated long read library preparation.
MIRO CANVAS is a digital microfluidics (DMF) platform that allows low throughput workflow automation for complex protocols, such as NGS library preparation. The system
is compatible with a wide range of reagents. This application note describes the results that can be expected when using the SMRTbell Prep Kit 3.0 in a protocol developed for the MIRO CANVAS.
The resulting research use only libraries can then
be sequenced using the PacBio sequencing systems.
Key benefits
Whole genome sequencing (WGS) library • This protocol offers the flexibility to choose preparation with PacBio's SMRTbell Prep automated, fast bead-based size selection, Kit 3.0 is fully automated on the or a more stringent gel-based size
MIRO CANVAS using 1-3 µg of high quality, selection. high molecular weight input DNA.
For the SMRTbell Prep Kit 3.0 protocol, MIRO CANVAS total library quantities, peak sizes and primary sequencing metrics are indistinguishable from manually prepared libraries.
Overview: How to automate PacBio SMRTbell WGS on MIRO CANVAS
Experimental set-up
The SMRTbell Prep Kit 3.0 protocol was designed with automated systems - such as the MIRO CANVAS - in mind, and has been tested using high quality, high molecular weight 1-5 µg DNA inputs. Before beginning, DNA should be fragmented to 15-18 kb using a Megaruptor®, and quantified before and/or after fragmentation using a broad range Qubit
quantification kit or similar. For the SMRTbell Prep Kit 3.0, post-shearing clean-up, repair and A-tailing, adapter ligation, post-ligation clean-up, nuclease treatment and bead-based size selection are all automated on MIRO CANVAS (Figure 1), resulting in a ready-to-sequence library.
4 H 30 MIN
15 MIN
SMRTbell Prep Kit 3.0
Hands-on
Repair & A-tailing • Adapter ligation & clean-up
time
Nuclease treatment AMPure® PB bead size selection
Figure 1: Experimental set-up. For the SMRTbell Prep Kit 3.0, the MIRO CANVAS automates all of the steps following reaction set-up.
Results
SMRTbell libraries were constructed with 1-5 µg of high quality, high molecular weight NA24385 (HG002)* DNA. Final library quantities for both manually-prepared and
MIRO CANVAS-prepared libraries were assessed using broad range or high sensitivity Qubit kits. For each of the kits evaluated, the MIRO CANVAS produced comparable libraries to manual preparation (Table 1).
* NA24385 DNA was obtained from the NIGMS Human Genetic Cell Repository at the Coriell Institute for Medical Research.
MIRO CANVAS
159 16
Manual
18 16
Input DNA
1 μg
SMRTbell Prep Kit 3.0
Total library (ng)
Table 1: SMRTbell Prep Kit 3.0 libraries generated with the MIRO CANVAS were comparable to manually prepared libraries. Total library quantity shown as average +/- standard deviation (n=6).
Efficient removal of small molecules from libraries using diluted bead-based size selection with the SMRTbell Prep Kit 3.0
Diluted bead-based size selection offers many advantages over traditional gel-based size selection of long read libraries, including automatability, reduced workflow times, and lower input requirements. Performing the SMRTbell Prep Kit 3.0 protocol on the MIRO CANVAS enabled construction of libraries from just 1 µg of input material, and efficiently removed small molecules from the library with automated bead-based size selection (Figure 2).
LM
17167
16000
15000
14000
13000
12000
11000
10000
9000
8000
7000
6000
5000
4297
Figure 2: SMRTbell Prep Kit 3.0 library size distribution. Following automated preparation on the MIRO CANVAS, including bead-based size selection, libraries were examined with a Femto Pulse (Agilent) to demonstrate efficient removal of small molecules.
SMRTbell libraries were sequenced on a Sequel® II System using Binding Kit 2.2, Sequencing Kit 2.0, and 30 hour movies. This demonstrated equivalency across manual and automated library preps. Of particular note, the new SMRTbell Prep Kit 3.0 not only requires less input material and eliminates the need for cumbersome gel-based size selection methods, it also results in libraries with excellent sequencing performance (Figure 3) and structural variant detection (Figure 4).
135,000
120,000
105,000
90,000
75,000
60,000
45,000
30,000
15,000
0
HiFi read length distribution
HiFi read quality (median)
Q37
13,035 HiFi read length
(mean, bp)
29,567,143,518 HiFi yield (bp)
HiFi reads
2,268,270
Analysis metrics
Value
0 5,000 10,000 15,000 20,000 25,000 30,000
HiFi read length, bp
Figure 3: SMRTbell Prep Kit 3.0 sequencing metrics. MIRO CANVAS yield, read length and read quality metrics are all equivalent to manually prepared libraries.
25,000
20,000
15,000
10,000
5,000
0
Miro Manual
Deletion Insertion Translocation Duplication Inversion
MIRO CANVAS
Manual
Deletion
9,070
9,174
Duplication
446
442
Insertion
12,586
12,437
Inversion
88
94
Translocation
122
162
Total
22,312
22,309
Figure 4: SMRTbell Prep Kit 3.0 structural variant detection. MIRO CANVAS detection of deletions, duplications, insertion, inversions and translocations in a NA24385 (HG002) DNA sample are all comparable to manually prepared libraries.
Conclusion
MIRO CANVAS is an advanced DMF platform that can be used to automate library preparation with the PacBio SMRTbell Prep Kit 3.0.
When using the SMRTbell Prep Kit 3.0 on the MIRO CANVAS, the protocol is fully automated from post-shear clean-up to elution.
Both MIRO CANVAS and manual library preparation yield high quality libraries with comparable sequencing performance and structural variant detection.
For more information and a list of materials used, please refer to our website.
Automating the Oxford Nanopore Ligation Sequencing Kit on MIRO CANVAS®
Efforless long read sequencing library prep
Long read sequencing is particularly well suited for detection of large genomic mutations, coverage of long repeat regions that confound short read assemblies,1 and identification of signatures that can be lost due to PCR amplification (including relative abundance in metagenomic samples2 and nucleotide modifications present on original DNA).3
Oxford Nanopore Technologies (ONT) long read sequencing of single-stranded DNA and RNA moving through nanoscale pores has been a major technological achievement in genomic research.3,4 Its advantages include the use of a small, portable sequencer that can be deployed in the laboratory or the field, low capital cost requirements, rapid turnaround times, and a user- friendly bioinformatics pipeline that allows real-time analysis during sequencing.2
MIRO CANVAS is a digital microfluidics (DMF) platform that allows low throughput workflow automation for complex protocols, such as NGS library preparation.
The system is compatible with a wide range of reagents. This application note describes
the results that can be expected when using the ONT Ligation Sequencing Kit in a protocol developed for the
MIRO CANVAS. The resulting research use only libraries can then be sequenced using ONT sequencing platforms.
Key benefits
Library preparation using the 1D ONT Ligation Kit is fully automated on the MIRO CANVAS.
This protocol has been demonstrated on MIRO CANVAS using 1 μg of high quality, high molecular weight input DNA.
N50 comparable to manual library prep.
75 % reduction in reaction volumes compared to manual library preparation.
2 hr 30 min run time.
Overview: How to automate the ONT Ligation Sequencing Kit on MIRO CANVAS
Experimental set-up
The protocol was designed for fully automated use on the MIRO CANVAS, and has been tested using 1 μg of high quality, high molecular weight (HMW) input DNA. Before beginning, DNA should be quantified using a broad range Qubit quantification kit or similar. DNA repair and end prep, post repair bead clean-up, adapter ligation and library clean-up are all automated on the MIRO CANVAS (Figure 1). Downstream quantification requires additional hands-on time.
Hands-on time
15 MIN
ONT Ligation Sequencing Kit
2 HR 30 MIN
DNA repair & end prep • Post repair bead clean-up Adapter ligation • Library clean-up
Figure 1: Experimental set-up. The MIRO CANVAS automates all the steps following reaction set-up, including DNA repair and end prep, post-repair bead clean-up, adapter ligation and library clean-up.
Results
For both manual library preparation and libraries prepared on the MIRO CANVAS, 1 μg of ZymoBIOMICS HMW DNA standard was used as input. For both preparation types, volumes listed in the ONT Genomic DNA by Ligation Protocol were reduced by 75 %. Each prepared library was loaded into a MinION flow cell and sequenced for up to 3 hours. Libraries prepared using the automated workflow on the MIRO CANVAS produced comparable read length distributions (Figure 2) and N50 read lengths (Table 1) to those prepared using the manual technique.
Manual MIRO CANVAS
60Mb
50Mb
40Mb
30Mb
20Mb
10Mb
0b
1.02 kb 12.3 kb 23.6 kb 34.8 kb 46.1 kb 57.3 kb 68.6 kb 79.9 kb
Read length
40Mb
Passed basecalled basses
30Mb
20Mb
10Mb
0b
1.02 kb 11.3 kb 21.5 kb 31.7 kb 42.0 kb 52.2 kb 62.5 kb 72.7 kb 82.9 kb
Read length
Figure 2: Read length distribution of libraries prepared manually and using the MIRO CANVAS. Representative read length histogram shows a similar distribution for libraries prepared both manually and using MIRO CANVAS.
Table 1: Summary of the sequencing metrics for libraries prepared manually and using the
MIRO CANVAS. Representative sequencing metrics for libraries prepared manually and using the MIRO CANVAS are shown below. Read length and quality statistics are comparable between MIRO CANVAS and manual preparation.
SEQUENCING METRICS
MANUAL
MIRO CANVAS
Mean read length
6,051
5,981
Mean read quality
10.8
9.7
Median read length
1,663
1,756
Median read quality
11.4
10.1
Numbers of reads
253,463
217,098
Read length N50
23.71 kb
23.06 kb
Total bases
1.55 Gb
1.31 Gb
The five longest sequenced reads in the MIRO CANVAS library were all longer than 120 kb, and of similar length to the five longest ranked reads from the manually prepared library.
Additionally, the longest read from the MIRO CANVAS library exceeded the length of the longest read from the manually prepared library, and had a higher mean call base quality score (Figure 3).
180,000
Read length
170,000
160,000
150,000
140,000
130,000
120,000
110,00
Mean call base quality score
12
11
10
9
8
7
6
Run type
Manual
1
Five longest reads
2 3 4
5
MIRO CANVAS
Figure 3: Read length and mean call base quality score for the five longest reads sequenced.
The ZymoBIOMICS HMW DNA standard is composed of genomic DNA from seven bacteria and one yeast species. All of the eight expected species were identified through nanopore sequencing of libraries prepared both manually and using the MIRO CANVAS.
Figure 4 shows how the cumulative reads of each species compare between manual and MIRO CANVAS runs.
Manual
MIRO CANVAS
Salmonella Escherichia Enterococcus Staphylococcus Pseudomonas Listeria Bacillus
Shigella
83,067
52,641
20,011
11,849
8,362
8,015
6,334
2,134
Salmonella
51,982
Escherichia
35,669
Enterococcus
19,242
Staphylococcus
11,629
Pseudomonas
8,821
Listeria
8,816
Bacillus
7,732
Shigella
1,508
Saccharomyces
1,413
Saccharomyces
1,459
Figure 4: Cumulative read numbers for representative mock community using libraries prepared both manually and with the MIRO CANVAS.
Due to its compact nature, simple set-up and minimal infrastructure requirements (a 120V adapter), the MIRO CANVAS can support scientists outside of the laboratory and facilitate collaboration between working groups. The MIRO CANVAS has been tested after air travel in carry-on baggage and in a backpack (Figure 5).
Figure 5: The MIRO CANVAS's compact dimensions (20.2 cm W x 40.6 cm D x 17.6 cm H) make it easy to packed for travel, providing the ideal companion for ONT's portable sequencers.
Conclusion
The MIRO CANVAS is an advanced DMF platform that can be used to automate library preparation with the ONT Ligation Sequencing Kit.
The ONT Ligation Sequencing Protocol for the MIRO CANVAS is fully automated - from DNA repair step to elution - can reduce reagent volumes by 75 %, and yields results comparable to manual library preparation.
Furthermore, its portability and compatibility with standard electrical sockets make it an ideal companion for highly portable ONT sequencers, offering library preparation and sequencing beyond the walls of the traditional laboratory.
For more information and a list of materials used, please refer to our website.
Implementing the Twist Human Core Exome Kit on MIRO CANVAS®
Automated NGS library preparation and hybridization capture
Whole exome sequencing (WES) provides a unique opportunity to dive deeply into the coding regions of the genome. It plays an important role in generating data for research and, in some cases, clinical applications.¹ Strong WES analyses rely on even coverage of these regions and, ultimately on high quality capture reactions.2 Variability in coverage can be minimized
by ensuring that reagents are high quality, and by automating laboratory processes that have traditionally been performed manually.3
The Twist Human Core Exome kit undergoes thorough quality control (QC) testing to ensure that all probes in the probe pools are present at the appropriate levels in order to limit wasted reads.4 The uniformity of these reagents reduces costs and improves coverage of reads in singleplex and 8-plex pools.4 Automating this kit on the MIRO CANVAS reduces the potential for contamination and variability. MIRO CANVAS also provides full walk-away automation, giving users the flexibility to perform other tasks while maintaining high quality results.
MIRO CANVAS NGS prep system is a digital microfluidics platform that allows custom low-throughput workflow automation for complex protocols, such as next generation sequencing (NGS) library preparation and hybridization capture. The system is compatible with a wide range of reagents.5 This
application note describes the results that can be expected when using the Twist Human Core Exome kit in protocols developed for MIRO CANVAS. The resulting research use only libraries can then be sequenced using Illumina sequencing platforms.
Key benefits
Library preparation and hybridization • These protocols have been developed capture using Twist Human Core Exome kit using 50 ng DNA input for library prep and are automated on MIRO CANVAS. 1500 ng for hybridization capture.
Depth of coverage, quality scores and • Automating library preparation and other key metrics are comparable between hybridization capture on MIRO CANVAS manually prepared libraries and those run reduces hands-on time by over 85 %.
on MIRO CANVAS.
Overview: How to implement the Twist Human Core Exome kit on MIRO CANVAS
Experimental set-up
The fully automated Twist Universal Library Prep protocol has been tested using 50 ng of high molecular weight DNA. Before beginning, DNA should be quantified using the QubitTM dsDNA Broad Range Quantification Assay or similar.6 Fragmentation, end repair, adapter ligation, amplification and purification steps are all automated in this protocol. The Twist Fast Hybridization Target Enrichment protocol has been tested using single samples and 8-plex
15 MIN
Hands-on
3 HR 20 MIN
Miro Universal Library Prep (Twist)
Fragmentation • End repair • Adapter Iigation • Purification Amplification • Purification
pools, and the volume of each sample library used depends on their respective concentrations.7 Minimal hands-on sample preparation is required at the beginning of this protocol, leaving most steps automated on MIRO CANVAS, including hybridizing probes with pools, binding targets to beads, post-capture amplification and purification.
1 HR
Hands-on
5 HR 30 MIN
Twist Fast Hybridization Target Enrichment
Hybridize probes with pools • Bind targets • Washes
Amplification • Purification
Figure 1: Experimental workflows. Both the MIRO Universal Library Prep and Twist Fast Hybridization Target Enrichment protocols are fully automated after reaction set-up.
Results
Library preparation
The MIRO Universal Library Prep (Twist) protocol has been tested using 50 ng of NA12878* gDNA. To assess the quality of the library preparation protocol alone on the MIRO CANVAS, hybridization capture was then performed manually. 4 replicates each of the libraries prepared on MIRO CANVAS and manually, 8 in total, were sequenced on an Illumina NextSeq High Output 75PE platform. Key metrics, such as depth of coverage and Fold-80 scores, were comparable between the methods. Additionally, both methods yielded a similar percentage of duplicated reads and reads on target (Figure 2).
*NA12878 DNA was obtained from the NIGMS Human Genetic Cell Repository at the Coriell Institute for Medical Research.
Figure 2: Multiple metrics were used to evaluate the sequencing data generated using both manual and Twist Universal Library Prep protocols. Hybridization capture was performed manually. All samples were subsampled to 150x raw sequencing coverage (70M reads,
5.3 GB of data per sample).
Target enrichment
The Twist Fast Hybridization Target Enrichment protocol has been tested using inputs of both 1,500 ng of mixed DNA for 8-plex pools (multiplex, 187.5 ng per library), and 500 ng for individual libraries (singleplex). Samples run on MIRO CANVAS for hybridization capture previously underwent manual library preparation. Sequencing was performed using the NextSeq High Output 75PE platform. Samples that were run on MIRO CANVAS, both singleplex and 8-plex pools, were compared to singleplex samples that had been enriched manually. Coverage of target bases at 20x and 30x was comparable between manual and automated protocols for single samples and 8-plex pools. Fold-80 scores were also similar between methods, with median scores varying no more than 0.02 (Figure 3).
100 %
% Targeted Bases Covered with X Reads
95 %
90 %
85 %
80 %
75 %
70 %
65 %
Manual singleplex MIRO CANVAS singleplex MIRO CANVAS 8-plex
Depth of coverage
2.0
94.4 %
94.4 %
93.7 %
96 %
96.7 %
96.3 %
1.9
1.8
1.7
1.6
1.5
1.4
1.3
1.2
1.1
1.0
1.39
1.38
1.40
Manual singleplex MIRO CANVAS singleplex MIRO CANVAS 8-plex
Fold-80
20x 30x
Figure 3: Coverage of targets and Fold-80 scores did not differ significantly between manual singleplex, MIRO CANVAS singleplex, and MIRO CANVAS 8-plex. All samples are subsampled to 150x raw sequencing coverage (70M reads, 5.3 Gb of data per sample).
Additional sequencing was completed using two 8-plex pools, one prepared manually and the other on MIRO CANVAS. Targets covered at 30x and Fold-80 scores for each pool were comparable (Table 1). Reads for these runs were assessed using the MIRO CANVAS Integrative Genomics Viewer (IGV). The IGV outputs displayed confident mapping of target genes across multiple exons from pools enriched using MIRO CANVAS (Figure 4).
Table 1: Key metrics were comparable for 8-plex pools enriched manually and on MIRO CANVAS. All samples were subsampled to 150x raw sequencing coverage (70M reads, 5.3 Gb of data per sample).
METRIC
MANUAL 8-PLEX
MIRO CANVAS 8-PLEX
Fold-80
1.37
1.4
Off-bait
7 %
9.30 %
Mean coverage
58.4
72.4
Hs-library size
571M
443M
30x coverage
93 %
93.70 %
Zero coverage
1.10 %
1.30 %
AT dropout
6.88
5.71
GC dropout
0.64
0.74
Median insert size
248
200
Figure 4: Representative IGV browser tracks of EGFR exons 2-10 from core exome-enriched libraries prepared manually and on MIRO CANVAS.
Time savings
While the overall time taken between the library preparation and hybridization capture protocols does not vary significantly between manual preparation and MIRO CANVAS automation, the hands-on time is far less for the automated applications. Manual library preparation requires about 3 hours of hands-on time, while the Twist Universal Library Prep requires about
15 minutes to set up for a 3 hour 20 minute fully automated run.
The Twist Fast Hybridization Target Enrichment protocol begins with several steps that cannot be automated, such as preparing pools and hybridization mix, and therefore requires about an hour of benchtop work. The steps that follow are fully automated on MIRO CANVAS, taking
about 5.5 hours to complete. This hour of hands-on time pales in comparison to the time taken by the manual protocol, which requires as much as 7.5 hours of hands-on time.
Conclusion
MIRO CANVAS is an advanced digital microfluidics platform that can be used to automate library preparation and hybridization capture with the Twist Bioscience Human Core Exome kit. Both the Twist Universal Library Prep and Twist Fast Hybridization Target Enrichment protocols are fully automated from fragmentation to elution and hybridization to elution, respectively.
These automated protocols and their manual counterparts yield comparable results. However, the greatly reduced hands-on time required by MIRO CANVAS makes it a valuable tool for any laboratory.
For more information and a list of materials used, please refer to our website.
PCR clean-up protocol with Agencourt AMPure XP magnetic beads
Automatic magnetic bead purification with the VIAFLO 96 handheld electronic pipette
Agencourt AMPure XP magnetic beads (Beckman Coulter) are an efficient way to clean up samples for PCR, NGS, cloning and microarrays. The kit provides a solution for medium to high throughput requirements when carried out in a 96 well plate, but the protocol involves many washing
and transfer steps that make it tedious to perform manually. With the VIAFLO 96, a handheld 96 channel electronic pipette, multistep protocols such as PCR clean-up and DNA purification can be performed quickly and efficiently, increasing
throughput tremendously by transferring samples and reagents to all 96 wells at once. Thanks to its unique operating concept, the VIAFLO 96 remains as easy to use as a traditional handheld pipette and can even provide critical information (user-defined prompts) about the protocol steps.
Key benefits
The VIAFLO 96 enables transfer of • The partial tip loading of the VIAFLO 96 samples, reagents and wash solutions to allows purification of fewer than 96 DNA 96 wells at once, increasing the throughput samples if necessary; 8, 16, 24, 32, 40 of magnetic bead-based DNA purification or 48 GRIPTIPS® can be loaded for easy
methods. purification of different numbers of samples.
The optimal immersion depth for removing • The Tip Align setting of the VIAFLO 96 supernatant or adding liquid right onto automatically positions the tips in the center the samples is guaranteed by defining the of the wells of a 96 well plate, avoiding any z-height of the VIAFLO 96. disturbance of the beads.
Overview: How to automate PCR clean-up steps with VIAFLO 96
Experimental set-up
The VIAFLO 96 handheld electronic pipette with a three position stage (see Figure 1) is used to purify DNA with AMPure XP beads from Beckman Coulter. The following protocol is an example of a set-up for 96 samples, where each well of a 96 well plate is filled with 10 µl of DNA sample and 18 µl of AMPure XP beads, then further processed with the VIAFLO 96. The PCR purification can be performed manually or semi-automated using the VIAFLO 96 in automatic mode. Custom-made VIALINK programs are provided. The VIALINK programs are set up according to the manufacturer's protocol (AMPure XP Beckman Coulter).
Three position stage
Figure 1: Three position stage for VIAFLO 96.
Step-by-step procedure
Dispense AMPure XP beads into PCR tubes
Transfer AMPure XP beads from the stock solution into 12 PCR tubes placed in a cooling block from INTEGRA.
Note:
The cooling block is just used as a support in this instance, not for cooling down the samples.
To ensure a homogenous stock solution, beads are thoroughly mixed by shaking/inverting
until the solution appears consistent in color. The beads are transferred into 12 PCR tubes using the Repeat Dispense mode of a VIAFLO single channel 1250 µl electronic pipette. A customized VIALINK program (AMP_Transfer1)
is available to aid bead transfer. Figure 2: Transfer AMPure XP beads from the stock solution into
12 PCR tubes.
For optimal pipetting, ensure beads are thoroughly mixed before each transfer. Mixing steps can be defined by the number of cycles and the pipetting speed. Both influence the efficiency of mixing and thus the quality of the clean-up. Saving these parameters in the pipetting program
ensures that mixing is always carried out as defined, yielding consistent results. Insert a pre- and post-dispense step to enhance accuracy and precision while pipetting precious reagents, such as AMPure XP beads.
Tip:
The use of sterile, filter, low retention GRIPTIPS ensures that every dispense is as accurate as possible, with no loss of beads or sample.
Transfer AMPure XP beads into the DNA samples
Transfer AMPure XP beads from the PCR tubes into a 96 well plate preloaded with DNA samples.
Pipette the beads from the PCR tubes into the 96 well plate using a VIAFLO 12 channel 50 µl electronic pipette. For optimal pipetting, make sure the tips are exchanged, and mix the beads thoroughly before each transfer. A customized VIALINK program (AMP_Transfer2) is provided for this step.
Tip:
Use low retention GRIPTIPS to minimize loss of beads adhering to the tip wall.
Figure 3: Transfer AMPure XP beads from the PCR tubes into a 96 well plate preloaded with DNA samples.
Mixing and binding of the AMPure XP beads
Mixing and binding of the magnetic beads to the PCR samples.
Load GRIPTIPS (position A) then select and run the AMPure_XP_M program on the VIAFLO 96. The samples are now mixed 10 times by pipetting up and down on position B. A five minute wait time follows, timed by the VIAFLO 96, to allow the DNA to bind to the beads.
Tip:
Use the z-height setting of the VIAFLO 96 to define the optimal tip immersion depth. This prevents air entering the tip during mixing and
Figure 4: Mixing and binding of the magnetic beads to the PCR samples.
avoids the pipette tip touching the bottom of the plate. Setting the Tip Align support strength to 3 for positions A and B makes it more comfortable to use the VIAFLO 96. These settings can be incorporated into the program so that they are not forgotten.
Magnetic separation of the AMPure XP beads
Separating the magnetic beads from the PCR samples.
Note:
Make sure new GRIPTIPS are loaded before continuing the protocol to ensure removal of the supernatant without bead carryover.
A prompt on the pipette screen reminds the user to move the sample plate from position AB onto the 96 well magnet (position B) and place an automation friendly reagent reservoir for waste collection on position AB. After a two minute incubation time the beads form a ring-shaped structure and the solution becomes clear. Load new GRIPTIPS before continuing the procedure to ensure accurate removal of the supernatant
Figure 5: Separating the magnetic beads from the PCR samples.
without bead carryover. Follow the instructions on the pipette and aspirate the supernatant slowly from the sample, dispensing it into the waste reagent reservoir (position AB).
Tip:
To avoid disturbing the ring of beads the supernatant is aspirated slowly at speed 1. Leave
5 µl of supernatant in the plate to prevent beads being drawn out during aspiration. The z-height limit is again used to ensure that the beads are not disturbed during pipetting.
AMPure XP bead clean-up
Wash the magnetic beads twice with 70 % ethanol.
Place an automation friendly reagent reservoir containing 70 % ethanol on position A and change the GRIPTIPS before continuing with the wash step. Follow the prompts on the pipette.
Pre-wet the GRIPTIPS with 70 % ethanol. Then wash the samples with 70 % ethanol. Repeat the washing step again as indicated by the pipette.
Tip:
Pre-wetting the GRIPTIPS with 70 % ethanol ensures equilibration of the humidity and the temperature between the air in the pipette/tips
Figure 6: Wash the magnetic beads twice with 70 % ethanol.
and the sample/liquid. In-house testing has shown that low retention GRIPTIPS prevent ethanol from dripping while traveling from one pipetting position to another.
Elute samples from the magnetic beads
Elute the purified samples from the magnetic beads by adding the elution buffer.
As indicated by the pipette, replace the 70 % ethanol reagent reservoir on position A with an elution buffer reagent reservoir and move the sample plate from the magnet (position B) to position AB. Load new GRIPTIPS before continuing with the protocol. After transferring
and thoroughly mixing the elution buffer with the beads, the pipette prompts the user to place the sample plate back onto the magnet (position B). During the one minute incubation time, place a new 96 well plate on position AB.
Figure 7: Elute samples from the magnetic beads.
Transfer the sample eluates
Transfer the sample eluates into the new 96 well plate.
Note:
Load new GRIPTIPS to ensure a clean eluate transfer without bead carryover.
Continue with the same program, slowly and carefully transferring the eluates from position B into the new plate (position AB).
Tip:
Optimizing pipette settings (aspiration speed, volume and height) allows the volume of the transferred eluate to be maximized without
Figure 8: Transfer the sample eluates into the new 96 well plate.
carryover of beads. These settings can be easily tweaked at any time. Performing a test run with water before implementing any new assay is an ideal way to optimize pipette settings.
Remarks
Automatic mode
The VIAFLO 96 can also operate on its own, enabling less user interaction which in turn improves ergonomics and reproducibility. This also makes it even more ideal for use in tight spaces, such as under a laminar flow cabinet.
Partial tip load
If you are not working with a full set of 96 samples, the VIAFLO 96 is able to work with
any number of tips loaded, allowing purification of smaller numbers of samples.
Figure 9: Automatic mode and partial tip load.
Conclusion
The VIAFLO 96 is perfectly suited to magnetic bead purification in a 96 well format. An entire plate with 96 samples can be purified in a fraction of the time it would take with a traditional pipette.
Optimized tip immersion and pipette settings in combination with the use of low retention GRIPTIPS allow maximum sample recovery at the end of the purification protocol.
The VIAFLO 96 can guide the user through the entire protocol step by step, ensuring the correct workflow and enhancing the reproducibility of results.
The optional automatic mode of the VIAFLO 96 enables the instrument to operate on its own to minimize pipetting errors, making it even more ideal for use under a laminar flow cabinet.
For more information and a list of materials used, please refer to our website.
Beckman Coulter AMPure XP beads protocol for PCR purification
Automatic magnetic bead purification with ASSIST PLUS pipetting robot
Agencourt AMPure XP beads (Beckman Coulter) are used for DNA purification in a variety of applications, including PCR, NGS, cloning and microarrays. The ASSIST PLUS pipetting robot provides a solution for optimal bead separation and maximized recovery
of precious samples. User guidance throughout the entire protocol ensures an error-free pipetting procedure. Careful and accurate handling of the magnetic beads by the ASSIST PLUS leads to superior reproducibility and consistency during the experiment. Taken together,
Key benefits
The VIAFLO and VOYAGER electronic pipettes, in combination with
ASSIST PLUS, provide unmatched
pipetting ergonomics.
Optimal pipette settings, including tip immersion depth, pipetting speeds and angles, maximize reproducibility and
sample recovery.
Exact positioning of the pipette tips in the sample wells avoids the risk of disturbing the ring of magnetic beads or bead
carryover.
The ASSIST PLUS automates many steps of a magnetic bead purification protocol and guides the user through the remaining manual operations to ensure an error-free
process.
the ASSIST PLUS provides researchers with an easy and highly efficient way to purify DNA from PCR reactions using AMPure XP magnetic beads.
Overview: How to automate PCR purification steps with ASSIST PLUS
The ASSIST PLUS is used to purify DNA samples using AMPure XP beads (Beckman Coulter). The pipetting robot runs a VOYAGER 8 channel 125 µl electronic pipette with 125 µl sterile, filter, low retention GRIPTIPS®. The use of low retention GRIPTIPS guarantees optimal liquid handling of viscous (AMPure XP buffer) and volatile (70 % ethanol) solutions.
Below is an example set-up for 24 samples, preparing 10 µl DNA samples (position B) with
18 µl of AMPure XP beads (position A). The pipetting programs were prepared according to the manufacturer's protocol (AMPure XP, Beckman Coulter) using VIALAB software.
The protocol is divided into two programs that guide the user through every step of the PCR purification process.
Program 1: Binding (AMP_BINDING)
Program 2: Washing and elution (AMP_WASH_ELUTE)
Experimental set-up: Program 1
Deck position A:
PCR 8 tube strip containing the AMPure XP beads (Figure 1, blue), placed onto a cooling block from INTEGRA.
Note: the cooling block is just used as a support in this
instance, and not for cooling down the samples.
Deck position B:
96 well plate with 24 DNA samples for purification (Figure 1, green).
Deck position C:
96 well ring magnet.
VOYAGER 8 channel
125 µl
A B C
50/125 µl sterile, filter, low retention GRIPTIPS
PCR 8-Tube Strip on cooling plate - 200 µl
96 well plate Sapphire - 200 µl
96 well plate Sapphire on 96 well ring magnet - 200 µl
Figure 1: Pipetting schema, set-up for program 1.
Run program 1: Transfer & Binding
Select and run the AMP_BINDING program on the VOYAGER electronic pipette. The ASSIST PLUS pipetting robot immediately starts the protocol.
AMPure XP bead transfer
Transferring AMPure XP beads from an 8 tube PCR strip to a 96 well plate containing the DNA samples.
To ensure the AMPure XP buffer is homogenous, the beads are resuspended by pipetting up and down 10 times before being transferred to the samples. The beads and DNA fragments are thoroughly mixed together before the pipette automatically starts the timer for a five minute incubation period, ensuring optimal conditions for the DNA strands to bind onto the magnetic beads.
Tip:
Using low retention GRIPTIPS rather than regular GRIPTIPS prevents the loss of AMPure XP beads during the pipetting steps (see Figure 2).
Figure 2: The image highlights the advantages of using low retention GRIPTIPS versus regular GRIPTIPS when pipetting AMPure XP beads.
Figure 3: The beads and DNA fragments are thoroughly mixed together before the incubation.
Magnetic separation of the AMPure XP beads
Separating the magnetic beads from the PCR samples.
A message instructs the user to move the plate (position B) onto the magnet (position C). Continue the program to start the timer. After a two minute incubation on the magnet the beads form a ring in the sample well and the solution becomes clear. The program resumes automatically, and
the supernatant is removed. On completion of this step, the pipette prompts the user to continue with the AMP_WASH_ELUTE program and to replace the labware on position A with the 8 row polypropylene (PP) reagent reservoir containing the ethanol and elution buffer.
Tip:
The supernatant is aspirated slowly using the Tip Travel feature of the ASSIST PLUS to avoid disturbing the ring of beads. The Tip Travel feature keeps the tip immersion depth constant during aspiration and dispensing. 5 µl of supernatant remain in the plate to prevent beads being drawn out during aspiration.
Figure 4: The ASSIST PLUS settings allow removal of the supernatant without any bead carryover.
Experimental set-up: Program 2
Deck position A:
The 96 well PCR cooling block is replaced by an 8 row polypropylene (PP) reagent reservoir filled with 70 % ethanol in row 1 (blue) and elution buffer in row 2 (orange).
Row 8 is used for waste (purple).
Deck position B:
Emtpy 96 well plate.
Deck position C:
96 well ring magnet and 96 well plate with 24 DNA samples for purification (green).
VOYAGER 8 channel
125 µl
A B C
50/125 µl sterile, filter, low retention GRIPTIPS
8 row reagent reservoir 96 well plate Sapphire - 200 µl
96 well plate Sapphire on 96 well ring magnet - 200 µl
Figure 5: Pipetting schema, set-up for program 2.
Run program 2: Washing & Elution
Start the AMP_WASH_ELUTE program on the VOYAGER electronic pipette. The ASSIST PLUS washes the beads twice by automatically adding and removing ethanol.
Magnetic bead clean-up
Washing the magnetic beads twice with 70 % ethanol.
The programmed pipette settings allow the beads to be washed without disturbing the bead ring. At the end of the second washing step, all the ethanol is removed. If necessary, an additional drying time can easily be added using VIALAB software.
Tip:
The use of low retention GRIPTIPS prevents ethanol from dripping while traveling from position A to position C.
Figure 6: The image highlights the advantages of using low retention GRIPTIPS (left) versus regular GRIPTIPS (right) when pipetting ethanol.
Elute samples from the magnetic beads
Eluting the samples from the magnetic beads by adding an elution buffer.
The pipette prompts the user to move the reaction plate from the magnet (position C) to position B. Continuing the protocol, the ASSIST PLUS transfers the elution buffer to the DNA
samples bound to the magnetic beads (position B, orange). After mixing carefully and thoroughly 10 times, the pipette prompts the user to place the 96 well plate on the magnet (position C).
Transfer the sample eluates
Transferring the sample eluates into a new 96 well plate.
As indicated by the pipette, place a new 96 well plate onto position B and continue the program. The sample eluates are then transferred into the new plate automatically.
Tip:
Optimized pipette settings (aspiration speed, volume, height, tip travel and tip touch) allow the volume of eluate transferred to be maximized without carryover of beads (see Figure 7). A tip touch after the transfer removes droplets that may still cling to the end of the pipette tips. Pipetting heights on the ASSIST PLUS can be fine-tuned at any time. Performing a test run with water before implementing any new assay is an ideal way to optimize pipette settings.
a)
b)
Figure 7: Magnetic beads are clearly visible in the 96 well plate with no supernatant remaining (A). No carryover of beads is observed in the eluate (B).
Conclusion
Magnetic bead purifications can be easily automated on the ASSIST PLUS pipetting robot.
Optimized tip immersion and pipette settings together with the use of low retention GRIPTIPS allow maximum sample recovery at the end of the purification protocol.
The pipette loaded onto the ASSIST PLUS prompts the user when needed, eliminating the risk of human errors.
VIALAB programs can be easily adapted to specific labware.
Prolonged pipetting tasks lead to repetitive strain injury. This can be avoided by automating these steps with the ASSIST PLUS.
For more information and a list of materials used, please refer to our website.
Automated size selection with CleanNGS, the cost-effective alternative to AMPure XP
Proven NGS size selection protocols on the ASSIST PLUS pipetting robot
DNA size selection with magnetic beads plays a significant role in molecular biology, employing specific ratios to capture and separate fragments by size. After the polymerase chain reaction (PCR), single-sided DNA size selection uses 1 high magnetic bead ratio to remove primer dimers, ensuring PCR product purification for efficient downstream sequencing. In contrast, DNA double-sided size selection uses 2 ratios to effectively remove small and large fragments, resulting in the purification of specific target average fragment sizes. Both single- and double- sided DNA size selections with magnetic beads are integral to next generation sequencing (NGS) library preparation.
Level up your PCR clean-up protocol and DNA size selection workflow using magnetic beads with our fully automated size selection protocols. The VOYAGER adjustable tip spacing pipette on the ASSIST PLUS pipetting robot automates all
liquid handling steps and the MAG module ensures precise automated magnetic bead handling. The protocols provided demonstrate accurate handling of magnetic beads for reproducible PCR product purification and DNA size selection. Using CleanNGS and AMPure XP magnetic beads (Beckman Coulter Life Sciences) confirmed
their interchangeability, establishing CleanNGS as a cost-effective alternative offering reliable results.
Key benefits
Efficient automated magnetic bead handling • Efficient and reproducible PCR product when using the MAG module - our module purification for downstream sequencing for magnetic separation. MAG captures and uses CleanNGS magnetic beads and releases magnetic beads with vertical back removes fragments below 100 bp.
and forth magnet movement to the plate without user interference, avoiding possible
spillage during manual plate transfer.
Fail-proof liquid handling of magnetic beads with the VOYAGER adjustable tip spacing pipette on the ASSIST PLUS pipetting robot, thanks to optimized pipetting height
and speed settings in VIALAB.
Reduce the processing costs and boost your NGS library preparation reproducibility for double-sided DNA size selection with
CleanNGS magnetic beads.
Flexibility is key! No matter what labware you are using, MAG's adapter selection provides optimized solutions for automated magnetic bead handling in microcentrifuge
tubes, 96 and 384 well PCR plates.
Gain additional hands-free time with ASSIST PLUS and MAG and effortlessly adapt to various DNA size selection protocols. VIALAB's user-friendly programming makes adjusting magnetic
bead ratios easy.
Overview: How to automate DNA size selection with the ASSIST PLUS and MAG
In this application note, we demonstrate fully automated DNA size selection of 48 samples with CleanNGS magnetic beads on the ASSIST PLUS pipetting robot. The 8 channel 125 µl VOYAGER adjustable tip spacing pipette automates the liquid handling steps and the
MAG module automates the magnetic bead handling steps in this protocol (we provide a semi-automated workflow using a magnet plate on our website. Scan the QR code at the end of the article to access it.).
Figure 1 illustrates the step-by-step procedure of the provided size selection protocols for:
Single-sided DNA size selection (PCR product purification)
Double-sided DNA size selection
Single-sided size selection
Double-sided size selection
Figure 1: Step-by-step procedure of single- and double-sided DNA size selection.
DNA single-sided size selection (PCR product purification)
Bind PCR product to a 1.8x magnetic bead ratio
Prepare fresh 80 % ethanol, and bring CleanNGS magnetic beads up to room temperature (RT). Place a 96 well DWP in portrait orientation on position A with 450 µl of CleanNGS magnetic beads in wells A1-A8 (Figure 2, light blue), 1.6 ml of 80 % ethanol in wells B1-B8 (Figure 2, blue) and 320 µl of molecular grade water in wells C1-C8 (Figure 2, pink). Place one empty 96 well HardShell PCR plate on position B and one with 40 µl of sample in each well of the first half in landscape orientation on the MAG module with the 96 well adapter on
position C (Figure 2, yellow).
Select and run the VIALAB program 'MAG_PCR_product_purification'. With 125 µl sterile, filter, low retention GRIPTIPS®, VOYAGER on ASSIST PLUS will transfer 72 µl of magnetic beads from column A (Figure 2, light blue) of the DWP on position A (Figure 3a) to every well of the first half of the PCR plate on position C (Figure 3b). Magnetic beads are mixed 10 times before aspiration, and 15 times after dispensing into samples, guaranteeing a homogenous magnetic bead mixture. GRIPTIPS are changed automatically between samples. With
the magnet array disengaged (Figure 4a, position home, 0 mm), VOYAGER will initiate an incubation of 5 minutes at RT to bind the PCR fragments to the magnetic beads (Figure 4b).
Figure 2: Deck set-up for automated PCR product purification on the MAG module. Position A: Reagents - deep well plate (DWP) (light blue: CleanNGS magnetic beads; blue: 80 % ethanol; pink: molecular grade water). Position B: Target - 96 well HardShell® PCR plate. Position C: Source - MAG module with 96 well HardShell PCR plate with samples (yellow).
a) b)
Figure 3: VOYAGER on ASSIST PLUS transfers CleanNGS magnetic beads from (a) a 96 well DWP to
(b) a 96 well HardShell PCR plate on MAG with 96 well PCR plate adapter.
a) b)
Figure 4: MAG on ASSIST PLUS (a) without PCR plate adapter showing disengaged magnet array (position home, 0 mm) and (b) with 96 well PCR plate adapter and 96 well Hardhell PCR plate showing uncaptured magnetic beads.
Removal of small fragments and washing
MAG will automatically lift the magnet array from position home (Figure 4b) to position high at 29 mm (Figure 5a) and capture magnetic beads within 3 minutes (Figure 5b). The PCR plate adapter for MAG has small holes to visually confirm targeted capturing of magnetic beads at the well surface.
VOYAGER - using fresh GRIPTIPS for each sample - removes the supernatant while the magnet array remains engaged (Figure 5a). The pipette transfers the supernatant into columns F-H of the DWP on position A. Slow aspiration (speed 1) and precise height settings prevent magnetic bead loss during washing. Magnetic beads are washed twice with 125 µl of 80 % ethanol from columns B of the DWP on position A (Figure 2, blue). VOYAGER aspirates an additional time
to ensure complete removal of ethanol from each well. MAG will lower the magnet array by
5 mm (position low, 24 mm) followed by air drying for 3 minutes at RT. Lowering the magnet array before air drying will move the pellets closer to the well bottom, allowing easier elution and smaller volumes.
Tips:
The magnet step in VIALAB provides total control of the magnet array, by setting customized heights anywhere between 0 and 29 mm.
Worry-free liquid handling of ethanol uses fast aspiration and slow dispensing with a tip touch to prevent droplet formation.
a) b)
Figure 5: MAG on ASSIST PLUS (a) without 96 well PCR plate adapter showing engaged magnet array (position high, 29 mm), and (b) with 96 well PCR plate adapter and 96 well HardShell PCR plate showing captured magnetic beads after 3 minutes.
Elution of single-sided size selected fragments
40 µl of molecular grade water is then transferred from column C of the DWP on position A (Figure 2, pink) to every well of the first half of the PCR plate on position C. Mixing 25 times ensures proper resuspension of the magnetic beads, independently of the volume, followed by a 5-minute RT incubation for elution. Again, MAG will automatically lift the magnet array to 29 mm (Figure 5a) and capture magnetic beads within 3 minutes (Figure 5b). Afterwards, VOYAGER transfers 35 µl of eluate to the unused PCR plate on position B, leaving 5 µl in the plate at
position C to prevent magnetic bead carryover. At the end of the run, the user is prompted to store the PCR plate from position B, and remove the plate from the MAG.
DNA double-sided size selection
Binding sheared genomic DNA (gDNA) to a 0.7x magnetic bead ratio
The deck set-up for DNA double-sided size selection is similar to DNA single-sided size selection, but with 320 µl of CleanNGS magnetic beads (Figure 2, light blue), 350 µl of molecular grade water (Figure 2, pink), and 55 µl of sample in each well of the first half of a HardShell 96 well PCR plate (Figure 2, yellow).
Select and run the VIALAB program 'MAG_DNA_double_size_selection'. VOYAGER will follow the steps described in PCR product clean-up but transfers 38.5 µl of magnetic beads to each well containing a sample (Figure 3b). Mixing 10 times before every other aspiration using new GRIPTIPS before aspiration guarantees precise, low volume pipetting of magnetic beads.
Removal of large fragments (right size selection)
After capturing large fragments bound to magnetic beads (right size selection) (Figure 5b), VOYAGER will transfer 85 µl of supernatant from each well of the first half of the PCR plate to the corresponding well of the second half of the same plate on position C.
Binding target fragments to a 0.8x magnetic bead ratio, and removal of small fragments (0.8x, left size selection) during the washing process
Following the same procedure as the right size selection, MAG lowers the magnet array back to position home (Figure 4a) and VOYAGER transfers 5 µl of magnetic beads to the supernatant of the first size selection in the second half of the PCR plate (position C). A 5 µl pre-dispense guarantees accurate pipetting of small volumes of magnetic bead. The subsequent washing procedure mirrors the DNA single-sided size selection.
Elution of double-sided size selected fragments
MAG and VOYAGER will follow the same procedure used for DNA single-sided size selection but transfer 50 µl of molecular grade water before elution, and 45 µl after capturing magnetic beads with MAG (Figure 5b).
Tip:
Changing to different fragment sizes is trouble-free, as the operator can calculate the magnetic bead volume for any ratio, and simply update it in VIALAB.
Results
Most reagent kit providers for library preparation recommend AMPure XP magnetic beads for NGS. Here, we demonstrate equivalent performance of CleanNGS magnetic beads during full automation of single-sided DNA size selection using a 100 bp DNA ladder (Promega) to mimic PCR product purification and double-sided DNA size selection using sheared gDNA.
With the VOYAGER adjustable tip spacing pipette on the ASSIST PLUS pipetting robot, 48 replicates were processed using CleanNGS magnetic beads in rows A to D, and AMPure XP magnetic beads in rows E to H. Automated magnetic bead handling with optimized magnet array heights was ensured with the MAG module. The size-selected fragments (CleanNGS vs AMPure XP) were analyzed and compared using the 4150 TapeStation System (Agilent, complete data set can be found on our website. Scan the QR code at the end of the article to access it.).
Figure 6 illustrates the gel picture of row A (CleanNGS) and row E (AMPure XP) of the
96 well plate when performing single-sided DNA size selection with 100 bp DNA ladder and a
1.8x magnetic bead ratio. Both magnetic beads purified all fragments of PCR ladder smaller than 100 bp and ~70 % of 4 ng 100 bp fragments while recovering ~100 % of 65 ng fragments ranging from 200 bp to 1500 bp.
Figure 6: Single-sided DNA size selection with CleanNGS magnetic beads guarantees PCR product purification. Results of fragment analysis using a 4150 TapeStation showing a gel with 100 bp PCR ladder before (input) and after single-sided DNA size selection using a 1.8x ratio of CleanNGS (left,row A, n=6) or AMPure XP (right, row E, n=6).
Figure 7 illustrates an electropherogram (EPG) with 22 out of 24 replicates (outliers excluded) of CleanNGS (left) and AMPure XP (right) when performing double-sided DNA size selection of sheared gDNA using 0.8x-0.7x (left-right) magnetic bead ratios. Both magnetic beads achieved a similar recovery of over 12 % (n=22) of 330 ng sheared gDNA. Average fragment sizes
were 372 bp with CleanNGS and 396 bp with AMPure XP magnetic beads, while overall size variation for each reagent was below 5 %.
Figure 7: Efficient double-sided DNA size selection with CleanNGS magnetic beads. Results of fragment analysis using a 4150 TapeStation showing an electropherogram (EPG) of sheared gDNA before (*)
and after double-sided DNA size selection using a 0.8x-0.7x ratio (left-right) of CleanNGS (left, n=22) or AMPure XP (right, n=22).
Remarks
VIALAB software
The VIALAB programs can be easily adapted to your specific pipette, labware and protocols.
Partial plates
The pre-set programs offer laboratories complete flexibility to accommodate varying sample sizes, ensuring they can meet both current and future demands.
Semi-automation
A protocol that uses a magnet plate for a semi-automated workflow can be found on our website. Scan the QR code at the end of the article to access it.
Conclusion
Fully automated DNA size selection and PCR product purification uses the MAG module for reliable magnetic bead handling and the VOYAGER adjustable tip spacing pipette for precise liquid handling on the ASSIST PLUS pipetting robot.
Double-sided DNA size selection with CleanNGS magnetic beads is game-changing by reducing experimental costs. Fragments of 340 bp to 390 bp are successfully selected with 12 % recovery when using a 0.8x-0.7x (left-right) ratio. On top, CleanNGS results are comparable to AMPure XP, making them the cost-effective alternative.
Small fragment removal is stress free when performing PCR product purification with a 1.8x CleanNGS magnetic bead ratio, ensuring reproducible recovery of precious fragments above 100 bp.
Easy adjustment to different protocols with VIALAB's simple programming allows seamless adaptation to changing workloads by modifying magnetic bead volumes and magnet height settings, or sample counts.
For more information and a list of materials used, please refer to our website.
Automated DNA normalization for NGS library prep
Effortless normalization for 16S metagenomic Illumina sequencing
Next generation sequencing (NGS) is used for DNA and RNA sequencing in basic, applied and clinical research, as well as diagnostics. NGS was originally called 'massively parallel sequencing', referring to its capacity to analyze millions of sequencing reactions at the same time. At the start of the NGS workflow, libraries of similarly sized DNA fragments are created. Normalization of these DNA libraries is then performed, as differences in the concentrations of fragments affect the sequencing results, leading to under- or over-representation of individual fragments.
Normalization of nucleic acid concentrations - the equalization of sample concentrations - essentially involves dilution of the sample with varying volumes of diluent. Manual normalization is error-prone and tedious and requires cumbersome calculations and constant focus to ensure accurate
sample tracking.
The ASSIST PLUS pipetting robot can be paired with the D-ONE single channel pipetting module to provide an easy automated solution
for library normalization, ensuring error-free dilution calculations, consistent pipetting steps and precise liquid handling.
Key benefits
Automating the D-ONE single channel pipetting module on the ASSIST PLUS pipetting robot provides hands-off, secure processing of library normalization
with high accuracy and increased
reproducibility.
The dilution calculator in VIALAB eliminates
errors in dilution calculations.
High precision pipetting results are guaranteed across a wide dynamic volume range, thanks to automatic selection of the
correct pipette tip size.
Optimal pipetting height is achieved by the automatic liquid level detection (LLD).
There is no need to specify initial volumes, which makes the program set-up easier and faster.
A run report summarizes all diluent and sample transfer volumes, giving full traceability.
No need to input countless values into VIALAB, since worklists can be imported in a .csv format. The template file - with preprepared column tags - makes data
transfer even easier for the user.
Overview: How to perform sample normalization for Illumina 16S metagenomic sequencing
This application note describes the automation of DNA library normalization. The protocol is based on Illumina's 16S Metagenomic Sequencing Library Preparation Guide. After quantifying the DNA libraries, the DNA concentrations should be calculated in nM concentration (see a calculation example for 500 bp long amplicons in the DNA concentration calculation Microsoft Excel® sheet). For sequencing, the libraries should be diluted to a concentration of 4 nM.
The 'Normalization worklist' programming step allows the user to import a worklist with sample details and concentrations (Figure 1). The dilution calculator (Figure 2) in VIALAB automatically determines the correct volume of each sample and diluent based on the target concentration (4 nM) and target volume (50 µl). It also automatically selects the best-suited pipette tip size.
Figure 1: Worklist template for the VIALAB program. Figure 2: Dilution calculator in VIALAB program.
Step-by-step procedure
The ASSIST PLUS pipetting robot is used together with the 0.5-300 µl D-ONE single channel pipetting module and 12.5 µl and 300 µl sterile, filter GRIPTIPS® to automate the pipetting steps of sample normalization for Illumina 16S metagenomic sequencing (Figure 3).
Figure 3: D-ONE single channel pipetting module is used to automate sample normalization.
The normalization protocol consists of two steps:
Step 1: Transfer of the diluent
Step 2: Transfer of the samples
Experimental set-up
Deck position A:
Diluent - 10 ml multichannel reagent reservoir
Deck position B:
Source plate - Sapphire 96 well PCR plate (Greiner Bio-one)
Deck position C:
Target plate - Sapphire 96 well PCR plate (Greiner Bio-one)
Figure 4: Deck set-up for sample normalization. Position A: Diluent - 10 ml multichannel reagent reservoir. Position B: Source plate - Sapphire 96 well PCR plate. Position C: Target plate - Sapphire 96 well PCR plate.
Transfer of diluent
The diluent is added in repeat dispense steps.
Place the 10 ml multichannel reagent reservoir on deck position A and fill it with 5 ml of 10 mM Tris (pH 8.5) buffer (Figure 4, blue with a white drop). Next, place the source plate (Sapphire 96 well PCR plate) with the amplicon samples on position B (Figure 4, colored circles) and an empty Sapphire 96 well PCR plate on position C (Figure 4, colored circles with dots); this will be the target plate containing the diluted, normalized samples.
Select and run the VIALAB program 'Illumina amplicon normalization'. The system first transfers the calculated diluent volumes to each well in several repeat-dispense steps with tip touch (Figure 5). A 15 µl pre- and post-dispense ensures precision while pipetting, and the total volume for each aspiration is calculated automatically. This is the fastest way of dispensing different volumes with a single channel pipette, saving time and money while reducing tip usage.
Tip:
Pre- and post-dispense settings, together with the tip touch, guarantee precise and accurate pipetting results.
Figure 5: The D-ONE single channel pipetting module transfers the required volume of diluent to each well.
Transfer of the samples
The samples are transferred one by one.
After dispensing the diluent, the ASSIST PLUS pipetting robot starts to add the amplicon samples from the source plate to the target plate in individual transfer steps (Figure 6).
The amplicon sample volumes in the source plate may be different. If the liquid level detection (LLD) (Figure 7) is activated, the optimal pipetting height is automatically detected and allows the pipette tip to follow the liquid level during aspiration. This constant submerge depth during aspiration contributes to precise pipetting results. At the end of every transfer step, the sample is mixed thoroughly with the diluent in three mixing cycles.
Tip:
Pipetting parameters (pipetting speed, pre-wetting, tip change) and the mixing conditions (height, speed, cycle and volume) can easily be adjusted in the VIALAB program if needed.
Now the normalized libraries are ready to be aliquoted and pooled for sequencing.
Figure 6: The D-ONE single channel pipetting module transfers the samples individually.
Figure 7: Optimal pipetting height is achieved in every well thanks to the automatic LLD.
Remarks
VIALAB software
VIALAB programs can be easily adapted to the user's specific labware and protocols.
Run report
If the ASSIST PLUS pipetting robot is connected to the PC with VIALAB, programs can be started directly from the PC. After the run, a run report is automatically generated,
documenting details such as the start/end time, user, calculated volumes, and any errors that occurred. This offers a convenient way to fulfill regulatory requirements.
Conclusion
The ASSIST PLUS pipetting robot equipped with the D-ONE single channel pipetting module is the perfect solution for DNA normalization for NGS. With the push of a button accurate, error-free sample dilutions can be achieved.
Several VIALAB features help the user to set up normalization quickly and easily. The dilution calculator determines the diluent and sample volumes, and automatically chooses the best pipette tip size to ensure perfect pipetting results. Worklists can be directly imported to VIALAB, saving the user from time-consuming, wearisome and error-prone typing.
The D-ONE single channel pipetting module performs automatic LLD to overcome the differing liquid levels of samples, ensuring the optimal aspiration height and precise pipetting.
Each D-ONE single channel pipetting module is compatible with two types of GRIPTIPS to provide a broad volume range. This ensures longer walk-away times and eliminates the need to change the pipette between pipetting steps.
For more information and a list of materials used, please refer to our website.
Affordable, high throughput bulk RNA barcoding and sequencing plate preparation with the VIAFLO 384
Streamline BRB-seq preparation with the VIAFLO 384
3' mRNA-seq is an effective tool for measuring gene expression in an unbiased and quantitative manner. However, existing technologies are too laborious and expensive to be applied to large scale projects with numerous samples. Alithea Genomics has developed a novel, proprietary technology called bulk RNA barcoding and sequencing (BRB-seq), which enables the streamlined preparation of 3' mRNA-seq libraries for hundreds of RNA samples in a single tube. The cornerstone of this technology is the use of BRB-seq oligos, which are synthetic DNA oligonucleotides.
BRB-seq oligos prime the reverse transcription (RT) reaction, during which the unique molecular identifier (UMI) and the sample-specific barcode are integrated into the synthesized complementary DNA (cDNA) strand. The use of BRB-seq oligos enables the molecular 'tagging' of individual RNA samples. After this initial tagging step, all samples (up to 384) can be pooled and processed simultaneously in a single
tube for the rest of the workflow.
Alithea Genomics provides kits that contain all the reagents necessary to perform BRB-seq on different sample types, including purified RNA, whole-blood RNA, and cell/organoid lysates.
The combination of the BRB-seq kits with the VIAFLO 96 and VIAFLO 384 systems provides a convenient, efficient
and reliable workflow for massively multiplexed RNA-seq experiments.
Key benefits
The BRB-seq workflow requires a very precise pipetting system, such as the VIAFLO 96 or VIAFLO 384, for
simultaneous transfer of small volumes of 96 or 384 samples from one plate to another.
Processing thousands of samples for BRB- seq can be drastically accelerated by using the VIAFLO 96 or VIAFLO 384 handheld electronic pipettes.
The 96 and 384 channel pipetting heads are interchangeable on the VIAFLO 384 handheld electronic pipette, providing the user the flexibility to transfer 96 or 384 samples in a single step.
High quality GRIPTIPS® provide a perfect tip seal. This guarantees that all tips on the VIAFLO 96 or VIAFLO 384 are at exactly the same height and will never fall off accidentally. This ensures increased accuracy and precision for liquid handling steps.
Overview: How to prepare samples for BRB-seq
Early-stage multiplexing is possible because of the highly optimized sets of barcoded primers (BRB-seq oligos), which are added during the RT reaction (Figure 1).
Figure 1: The barcoded oligo-deoxythymidine (dT) is attached at the RT step.
Step-by-step procedure
Either the VIAFLO 96 or VIAFLO 384 can be used for the main pipetting steps during sample preparation for BRB-seq. The instruments are equipped with a 96 or 384 channel pipetting head (0.5-12.5 µl) and a three position stage (with positions A, A/B, and B). 12.5 µl sterile, filter GRIPTIPS are used. Pre-washed cells (in a 96 well or 384 well Sample plate) are lysed with
10 or 5 µl cell lysis buffer (respectively). Customized VIALINK programs are provided for the cell lysate transfers and pooling steps (Figure 2, steps 2, 3 and 7) in 384 well plates. The custom VIALINK programs for 96 well plates are available in the download section.
Overview of the programs
Program 1: Transfer the cell lysates to the Stock plate - Cell lysate transfer Program 2: Transfer the cell lysates to the Barcode plate - Barcoding Program 3: Pooling of samples after RT reaction - Pooling
Figure 2: The sample preparation workflow for cell lysate BRB-seq of 384 samples (green arrows represent the transfer steps performed on the VIAFLO 384).
Cell lysate transfer
Transfer of cell lysates to the Stock plate.
Load a full box of 12.5 µl sterile, filter GRIPTIPS onto the VIAFLO 384. Slide the three position stage to the left so that it is in the second position (A/B-B). Launch the custom program '384_LYS_STORAGE'. A prompt on the pipette screen informs the user that the Sample plate (384 well cell culture plate, Corning) should be placed on position A/B, and the Stock plate - a new 384 well PCR plate (Eppendorf) placed on 384 well cooling block - on position B (Figure 3). The pipetting head should then be lowered and the pipette tips should be placed into the individual wells of the Sample plate. The pipette aspirates 7 µl of cell lysate from the wells of the Sample plate and dispenses it to the wells of the Stock plate. When the dispense step is finished, if more samples are to be transferred, the pipette will notify the user that the pipette tips need to be changed and the program restarted. Alternatively, if there are no further samples to transfer, the pipette will notify the user that the program is finished. The Stock plate can then be kept at -80 °C for later analysis or used immediately for continuation of the workflow.
Tips:
The aluminum 384 (or 96) well PCR cooling block keeps the samples or reagents chilled.
The Z-heights are defined in the programs to ensure optimal tip immersion depth.
Figure 3: The three position stage of the VIAFLO 384 set up for the cell lysate transfer step.
Barcoding
Transfer of cell lysates to the Barcode plate.
Load a full box of new 12.5 µl sterile, filter GRIPTIPS onto the VIAFLO 384. Move the three position stage to the second position (A/B-B). Select the custom program '384_LYS_OLIGOS'. The instrument will display the prompt that the Stock plate (on a 384 well cooling block) needs to be placed on position A, and the Barcode plate (on a 384 well cooling block) on position B. The pipetting head with the tips should then be lowered into the individual wells of the Stock plate (Figure 4). The VIAFLO 384 aspirates 5 µl of the cell lysate from each well of the Stock plate, and dispenses it to the Barcode plate that contains the optimized barcoded oligos. After the dispense step, if new cell lysates are to be transferred to new Barcode plates, the pipette informs the user that the pipette tips need to be changed. Alternatively, if there are no further samples to transfer, the pipette informs the user that the program is finished.
After adding the cell lysate to the barcodes, 10 µl of RT mix should be added manually with a 16 channel (12 channel in the case of 96 well plate) VIALFO pipette from PCR strip tubes (Starlab). The Reaction plate containing the cell lysates, the barcodes, and the RT mix needs to be transferred to a thermal cycler and incubated at 50 °C for 30 min.
Figure 4: The three position stage of the VIAFLO 384 set-up for the barcoding step.
Pooling
Pooling of samples after RT reaction.
After incubation, the barcoded cDNA samples can be pooled together. Load a full box of new
12.5 µl sterile, filter GRIPTIPS, slide the three position stage to the second position (A/B-B), and start the custom program '384_POOLING'. The pipette tells the user to place the Reaction plate (on the cooling block) on position A, an INTEGRA 150 ml automation-friendly reservoir on position B, and to lower the pipetting head with the tips into individual wells of the reaction plate (Figure 5). The pipette then aspirates 10 µl solution from each well, and dispenses it to the reservoir.
Figure 5: The three position stage of the VIAFLO 384 set-up for the pooling step.
Collecting the pool
Transferring the pool to a vessel.
The pool can be transferred to a 15 ml centrifuge tube (Greiner Bio-one) for further precleaning. This process can be easily executed with INTEGRA's PIPETBOY acu 2. The pool is ready for subsequent library preparation and next generation sequencing.
Results
Sample results obtained using either the BRB-seqTM kit, or the Cell lysate BRB-seqTM kit, and the VIAFLO 384 channel handheld electronic pipettes are shown below.
Figure 6: Distribution of the number of detected genes across 384 samples processed in one tube, using Alithea's MERCURIUS™ BRB-seq kit. The library was sequenced at an average of 1 million reads per sample.
Figure 7: Distribution of the number of detected genes across 384 samples processed in one tube, using Alithea's MERCURIUS™ cell lysates BRB-seq kit. The library was sequenced at an average of 1.5 million reads per sample.
Conclusion
'Large scale' and 'high throughput' are often synonyms of bulky, expensive, and fully automated liquid handling solutions. By combining Alithea Genomics' BRB-seq technology with the VIAFLO 96 or VIAFLO 384, we demonstrate that there is an easier way to achieve a reliable, ultra-high throughput and cost-efficient RNA-seq workflow.
With BRB-seq, up to 384 RNA or cell lysate samples can be processed in a single tube, right from the beginning of the library preparation workflow. With the VIAFLO 96 and 384 systems, the large number of pipetting steps can be condensed into handful of semi-automated plate- to-plate transfers.
Setting the Z-height and the Tip Align on the VIAFLO 96 and VIAFLO 384 minimizes pipetting variability.
Thanks to the compact benchtop design, both the VIAFLO 96 and VIAFLO 384 can fit into most laminar flow hoods, or on a small bench.
Large scale RNA-seq is becoming an important tool that biotech, pharma and academic institutes can use to discover and develop new drugs. Performing this large-scale RNA-seq using BRB-seq and the VIAFLO 96 or VIAFLO 384 drastically reduces the turnaround times and operational costs.
Using the BRB-seq kits on the VIAFLO 96 or VIAFLO 384 offers a convenient, compact and cost-effective solution to unlock the power of large scale RNA-seq.
For more information and a list of materials used, please refer to our website.
CHAPTER 4:
Customer testimonials
We are extremely proud of how INTEGRA liquid handling solutions are being used across the world to support scientific findings, from developing high throughput sequencing methods to solving the canine epigenome. In this chapter, we share a selection of testimonials from our customers, as they explain how these cost-effective instruments have helped to streamline and scale their research.
ASSISTing leukemia research at the Cancer Institute
Scientists are increasingly looking for solutions to improve throughput and time to results, which is why many are turning to INTEGRA's unique electronic pipette range - such as the ASSIST PLUS pipetting robot - to simplify their workflows. Yvette Hoade, a research technician in the Payne Laboratory, Department of Haematology, at University College London's Cancer Institute, is investigating mutations associated with different blood diseases.
From blood to genotype
Yvette's group is focused on uncovering the risk factors and mechanisms that give rise to bone marrow failure and myelodysplastic syndrome (MDS), both age-related disorders that greatly increase the risk of developing leukemia.
The lab has recently started a DNA extraction project, and is using VOYAGER adjustable tip spacing electronic pipettes to help streamline the process of pipetting between different labware types.
The ability to change tip spacing at the push of a button is ideal for this workflow, Yvette explained: "We've started this new project with the aim of quickly going from blood to genotype for a large batch of samples. We already had an 8 channel VOYAGER, which we use for loading gels, but decided to buy a larger volume 6 channel VOYAGER to help with this project. It's fantastic for speeding
Photo courtesy of University College London's Cancer Institute
up the sample extraction process, transferring 200 µl of blood from collection tubes into a 96 well format accurately and efficiently, and we then continue to use the VOYAGER pipettes for the rest of the DNA isolation protocol. Having both the high and low volume VOYAGER pipettes means that any reformatting we need to do is a breeze - we've got all volumes covered."
Automate your electronic pipette
After being impressed with the VOYAGER, the team looked to expand their range, and another product caught their collective eye. Yvette continued: "After speaking to the INTEGRA sales rep, we arranged a couple of demos of the ASSIST PLUS pipetting robot, and quickly realized how beneficial this would be to the group; it's such a versatile platform and could be used for so many of our applications. The VOYAGER can be mounted onto the system, which then automatically carries out our pipetting tasks according to our preprogrammed settings; it's
so much more efficient than manually pipetting a large batch of samples. The interface of the VIALAB pipetting automation software is clear and intuitive - anyone who wants to use the ASSIST PLUS can just set up their own protocol pretty easily. You can still use the pipette separately if you want to, so we can take the VOYAGER off and manually prepare samples if needed. It's amazing to have that flexibility in such a busy lab. We originally bought the ASSIST PLUS for the DNA extraction project, but now that we have it in our lab, we find that we're using it for everything, it was certainly worth the investment."
Higher throughput and increased accuracy
An efficient and reliable workflow is compulsory for Yvette's busy lab, and INTEGRA pipettes fit seamlessly into various aspects of their work, from start to finish. "Once we've extracted the DNA, we prepare libraries using PCR for next generation sequencing to screen for mutations that can predispose individuals to MDS or leukemia. The ASSIST PLUS is great for pipetting really small volumes, which means that we can now use 384 well plates and process more samples. We haven't been able to use these plates previously but, with the accuracy of the ASSIST PLUS, we have the confidence that our results will be precise and reproducible. It also really speeds up the whole process, which makes it less tedious, and reduces the chances of people making mistakes! We've got about 6,000 samples to sequence for this project, which is why we wanted a more automated process, and our throughput has been improved infinitely."
The perfect lab partner
"We've recently started looking at using the ASSIST PLUS for magnetic bead DNA clean up before pooling and sequencing our samples. Automation of this process will be a real
revelation, as clean ups are quite a hassle to do at the moment. INTEGRA has been great at offering advice on protocol development, and the customer support is fantastic. Any ideas we've had, such as suggestions for software updates, the team has been on board with; you really feel like you're being listened to and supported."
"We are incredibly happy with our experience with INTEGRA and its range of products. The level of automation provided by the ASSIST PLUS has been hugely beneficial to our entire group, and it can be used in many applications within our workflow. Automation gives us the confidence that the level of accuracy is high, and cuts out the inevitable tedious and repetitive aspects of lab work that comes with handling such large sample numbers. The ASSIST PLUS is the ideal lab partner, and there's often a queue to use it - it's a solid member of the team now," Yvette concluded.
Precise and flexible pipetting for genetic analysis and molecular biology applications
Microsynth, founded in 1989, is a leading European company in nucleic acid synthesis, analysis and sequencing. It offers high quality products and services for molecular biologists who require DNA/RNA oligonucleotides, robust sequencing - such as high throughput Sanger sequencing - and analysis solutions, as well as outsourcing of contract research projects.
Microsynth is using INTEGRA's MINI 96 and VIAFLO 96 handheld electronic pipettes in every step of sample preparation, in workflows ranging from nucleic acid isolation to next generation sequencing (NGS) and different types of PCR. Dr Sergey Yakushev, Head of Genetic Analysis at Microsynth, explained: "My department focuses on genetic analysis, using a range of methodologies - such as conventional PCR, sequencing, and DNA and RNA isolation - which require multiple liquid handling steps during sample preparation and analysis. We receive as many as 20,000 samples per year from our customers, including blood, tissue, pharmaceutical products and environmental substances. This high throughput is made possible with the use of multiple INTEGRA products, from single and multichannel manual pipettes to the MINI 96 and VIAFLO 96. We also have low and high volume VOYAGER adjustable tip spacing pipettes, which we use for multichannel pipetting between different labware formats."
Electronic pipettes for every application
Digital PCR is an expensive method that starts with adjustment of the analyte concentration in 96 well plates, a process that requires highly precise and accurate pipetting of samples. Dr Yakushev continued: "If there is pipetting imprecision, it becomes apparent very quickly in our results and, unfortunately, increases costs in terms of both time and money, as we need to repeat all the steps again from the beginning. In
qPCR, we replicate low volume samples
Photo courtesy of Microsynth
from 96 to 384 well plates, which typically involves multiple aspiration and dispense steps. Previously, we did this using a 96 channel pipette from another manufacturer, but this didn't match our expectations. We now use the MINI 96 for digital PCR and qPCR preparation, as well as in end-point PCR reactions and library preparation for NGS. All these applications require precise, simultaneous transfer of multiple liquid samples, and the small footprint, cost effective MINI 96 has allowed us to do exactly this. We use all the electronic features, including the defined pipetting heights, which is especially helpful when working in white, 384 well qPCR plates, where the wells are not transparent. Best of all, we get more hands-off time."
"The heterogeneity of the samples, special buffer conditions and liquid properties in DNA and RNA isolation workflows are not always compatible with liquid handling platforms. Manual and visual control is critical, which is why we use handheld pipettes. The VIAFLO 96 combines the advantage of being able to maintain manual processing for these workflows - moving easily between plates and reservoirs - with highly precise and repeatable pipetting into all wells of a 96 well plate simultaneously. For example, in cfDNA isolation, we use the VIAFLO 96 to pipette multiple samples with different viscosities over a wide volume range, which would otherwise be difficult to program on automated systems. Being able to keep a close eye on the speed of liquid aspiration and dispensing helps avoid any loss and reduces the likelihood of poor sample recovery, ensuring that quality standards are matched, and expectations are met."
The unmatched benefits of INTEGRA products and services
"The MINI 96 and VIAFLO 96 were purchased to upgrade the throughput and speed of liquid handling, to ensure high technical precision, workflow flexibility and comfort of lab work.
What also convinced us was the quality of design - even the plastic consumables such as the GRIPTIPS® pipette tips it uses - and the integrated technology that makes pipetting protocols smart. We can process up to 96 samples at once with the MINI 96 and VIAFLO 96, increasing the rate of highly accurate and precise pipetting and, therefore, throughput, all while decreasing the risk of unplanned costs due to sample loss and repeat analyses. In my opinion, INTEGRA handheld electronic pipettes are impressive as they solved the complications in our lab, and I truly believe they outperform 1, 8 and 12 channel pipettes."
"We're also very satisfied with INTEGRA's customer service, from the purchase and installation of the pipettes to coming on site for assistance and training. We were given a clear demonstration of how to operate and troubleshoot the equipment despite it being intuitive and everything being well presented in the manuals. Any time we had a question, we received a quick answer - INTEGRA has helped us a lot, and I love the pipettes!" Dr Yakushev concluded.
VIAFLO 96 and VIAFLO 384 used to provide high throughput and cost-effective
BRB sequencing
Alithea Genomics is a Swiss pioneer of fast, high throughput and robust bulk RNA barcoding and sequencing (BRB-seq) for large academic and pharmaceutical partners. The company originally began using VIAFLO 96 and VIAFLO 384 handheld electronic pipettes for its in-house BRB-seq service, but was so impressed with the throughput and precision that it is now using the instruments to prepare kits for customers to run their own sequencing protocols.
Alithea Genomics was created in May 2020, with the mission of supplying a BRB-seq service that could process samples for a fraction of the cost and time of traditional RNA-seq on a large number of tissue and blood RNA samples. For high throughput transcriptomics, BRB-seq is designed to save both reagents and time by pooling and processing multiple samples in a single tube straight after the first reverse transcription reaction, rather than processing them individually, as is required for standard RNA-seq protocols. As a result, this method lends itself to large research projects, and allows scientists to economically process a very high throughput of samples.
The best of both worlds
Speed, throughput and cost savings are the greatest advantages of BRB-seq, so the company needed a pipetting instrument that reflected these qualities. Riccardo Dainese, CEO of Alithea, explained: "For us, the INTEGRA VIAFLO 96 and VIAFLO 384 handheld electronic pipettes provide the best balance between automation, pricing and throughput. Our technology only really requires high throughput, precise pipetting for plate-to-plate transfers, so we didn't need a big, expensive liquid handling robot. The VIAFLO 384 allows us to seamlessly transfer and quickly process thousands of samples, while still retaining the flexibility to change between 96 to 384 channel pipetting heads as often as required."
Riccardo continued: "This combination of productivity and flexibility is particularly important for our three main customer sectors:
academic researchers, biobank owners and pharmaceutical companies. Academic research often has to contend with limited funding, while the other two areas encompass large RNA sequencing projects with significant sample pools. In all instances, we need to minimize costs and preparation times. In addition, the bigger the project and the more samples there are to process, the more important it is to move
away from small, manual pipettes to reduce the Photo courtesy of Alithea Genomics
physical effort and strain. The VIAFLO 384 gives us everything we need to achieve our target throughput, without the hefty price tag of a large, automated liquid handling robot."
Precise pipetting
Alithea initially purchased VIAFLO 96 and VIAFLO 384 instruments for its in-house BRB-seq service, but has since extended its use of these pipetting systems to include the assembly and aliquoting of sequencing kit plates for shipment to customers. Riccardo continued: "Being able to guarantee the precision of plate-to-plate transfers has been so important - especially for preparing ready-to-use plates for our customers - because it translates directly into data accuracy and uniformity. The VIAFLO 96 or VIAFLO 384 can aspirate a defined volume,
then multi-dispense smaller aliquots, providing us with a much more convenient workflow than manual pipetting, while helping to guarantee precision. Another key feature is that it is straightforward to change the pipetting head, even for users that don't have much experience with 96-channel electronic pipettes, making the systems very user friendly. Of course, the
GRIPTIPS® are an added bonus too, as we have never had a problem with loose tips falling off."
Technician's best friend - solving the dog epigenome to advance oncology
Researchers in the College of Veterinary Medicine at Seoul National University are using vacuum aspiration systems from INTEGRA Biosciences to streamline their next generation sequencing (NGS) workflows for comparative oncology. These instruments are helping the team to perform finely controlled microvolume aspiration of organoid cultures, accelerating their efforts to use animal models for the study of animal and human diseases.
Accelerating canine cancer research
The Cho Lab, within the College of Veterinary Medicine, studies naturally occurring tumors found in domesticated dogs as part of its translational research, with the aim of advancing our understanding of cancer pathogenesis in both animals and humans. Veterinary medicine is lacking the huge volume of NGS data that has transformed human cancer research, and the Korean lab has published the first mapped epigenome of the domesticated dog in a bid to similarly expand the number of canine NGS datasets.1 They are now using this data to study cancer biology using organoids, enabling meaningful observations as part of a comparative medicine strategy.
Researchers within the Cho lab are using the VACUSAFE safe aspiration system and the VACUSIP portable aspiration system for various steps in cell culture management, sample extraction and NGS library preparation. Borris D. Aldonza, facility manager for the lab, explained: "Our core interest is studying the biological development of naturally occurring cancers in non-human mammals,
and our place within the College of Veterinary Medicine gives us access to many diverse samples. One of our daily tasks is microvolume aspiration, and the compact VACUSAFE and VACUSIP systems enable a finely controlled aspiration technique, providing the accuracy needed to support our NGS work, including
in our successful publication of the
first reference epigenome of the
domesticated dog."
Photo courtesy of the College of Veterinary Medicine at Seoul National University
Aspiration systems for efficient and accurate organoid maintenance
The Cho Lab is using organoid cultures to study how the dog epigenome changes during cancer progression. Borris continued: "We are generating organoids from cancer-stricken canine models to provide the missing link - the primary data - to enable more reliable, dog- centric treatment decisions, and more meaningful observations on comparison with human organoids. All our benchtop cell culture instruments - including the vacuum aspiration systems - are used in our organoid research, including cell culture, culture maintenance and basic sampling-related activities. I had previously worked with INTEGRA's vacuum aspiration systems and, when choosing the initial equipment to set up our facility, I knew that we wanted them for our cell culture space."
"Our previous aspiration system used a foot pedal to control aspiration, which meant we often unintentionally aspirated the organoids along with waste liquid. In contrast, the VACUBOY vacuum hand operator on the VACUSAFE features a finger-activated valve that precisely regulates vacuum flow, enabling sensitive fingertip control. The VACUSAFE also has multiple vacuum intensity settings that allow us to control the amount of suction force, which is ideal for the gentle handling of precious samples like our organoids. Since we can control the aspiration speed so well, the accuracy of aspirating microvolumes has markedly improved. The VACUSIP is perfect for daily microvolume aspiration tasks, as it allows us to aspirate any remaining liquid from the samples - which is simply unachievable by manual pipetting - and saves us a lot of time for our microplate- and PCR plate-based samples," he added.
Future expansion helped by INTEGRA's products
"We now plan to expand the lab, to increase our cell culture capabilities and organoid research projects, and we anticipate acquiring more aspiration systems from INTEGRA to aid this growth. In addition, the lab is keen to introduce more streamlined liquid handling systems to help manage our increasing workload. The MINI 96 portable electronic pipette will be a perfect candidate to support this, particularly as we aim to provide a wealth of drug screening data for thousands of small molecules against both canine- and human-derived organoids. INTEGRA's products have helped us to achieve great feats so far, and we plan to keep moving the field of comparative oncology forwards using this robust and reliable equipment," Borris concluded.
CHAPTER 5:
Conclusion
In this eBook, we have explored how INTEGRA Biosciences' efficient library prep and liquid handling solutions can enhance NGS workflows, highlighting key applications and best practices. Whether you're a seasoned researcher or new to the field, the insights shared here aim to enhance your understanding of NGS, helping to accelerate scientific advancements. For continued support and more in-depth resources, visit our website where we have plenty of additional resources and articles to guide you as you embark on your NGS journey.
CHAPTER 6:
References
DNA sequencing methods
National Human Genome Research Institute (2022). Human Genome Project. https://www.genome.gov/about-genomics/educational-resources/fact-sheets/human- genome-project
Mullin, E. (2022). The Era of Fast, Cheap Genome Sequencing Is Here. https://www.wired.com/story/the-era-of-fast-cheap-genome-sequencing-is-here
Maxam, A. M., Gilbert, W. (1977). A new method for sequencing DNA. PNAS, 74(2), 560-564.
https://doi.org/10.1073/pnas.74.2.560
Sanger, F., Nicklen, S., Coulson, A. R. (1977). DNA sequencing with chain-terminating inhibitors. PNAS, 74(12), 5463-5467.
https://doi.org/10.1073/pnas.74.12.5463
Heather, J. M., Chain, B. (2016). The sequence of sequencers: The history of sequencing DNA. Genomics, 107(1), 1-8. https://doi.org/10.1016/j.ygeno.2015.11.003
Arney, K. (2021). DNA sequencing: the next generation. https://geneticsunzipped.com/transcripts/2021/9/23/next-generation-sequencing
Mashayekhi, F., Ronaghi, M. (2007). Analysis of Read-Length Limiting Factors in Pyrosequencing Chemistry. Analytical Biochemistry, 363(2), 275-287. https://doi.org/10.1016/j.ab.2007.02.002
Nguyen, J. (2021). Pyrosequencing. https://apollo-institute.org/pyrosequencing
Illumina (n.d.). Explore Illumina sequencing technology. https://www.illumina.com/science/technology/next-generation-sequencing/ sequencing-technology.html
Illumina (n.d.). One Decade of Sequencing. https://www.illumina.com/science/technology/next-generation-sequencing/illumina- sequencing-history/decade-in-sequencing.html
Shendure, J., Balasubramanian, S., Church, G. et al. (2017). DNA sequencing at 40: past, present and future. Nature, 550, 345-353. https://www.nature.com/articles/nature24286
Chow, E. (2019). Next Generation Sequencing 1: Overview - Eric Chow (UCSF). https://www.youtube.com/watch?v=mI0Fo9kaWqo
Depalma, A. (2018). Sanger Sequencing: Still the Gold Standard? https://www.labmanager.com/insights/sanger-sequencing-still-the-gold- standard-1758
Khan Academy (n.d.). DNA sequencing.
https://www.khanacademy.org/science/ap-biology/gene-expression-and-regulation/ biotechnology/a/dna-sequencing
Thermo Fisher Scientific (n.d.). What is Sanger sequencing? https://www.thermofisher.com/ch/en/home/life-science/sequencing/sequencing- learning-center/capillary-electrophoresis-information/what-is-sanger-sequencing.html
Weaver, N. (n.d.). Application of Magnetic Microspheres for Pyrosequencing on a Digital Microfluidic Platform. https://ece.duke.edu/sites/ece.duke.edu/files/Weaver_Rpt2005.pdf
Qiagen (n.d.). Pyrosequencing Technology and Platform Overview. https://www.qiagen.com/us/knowledge-and-support/knowledge-hub/technology-and- research/pyrosequencing-resource-center/pyrosequencing-technology-and-platform- overview
Gallego, A. (n.d.). How to break up DNA for NGS library prep. https://goldbio.com/articles/article/how-to-fragment-DNA-for-NGS
Illumina (n.d.). Processing more samples in less time. https://emea.illumina.com/techniques/sequencing/ngs-library-prep/multiplexing.html
Pacific Biosciences (n.d.). Sequencing 101: from DNA to discovery - the steps of SMRT sequencing.
Pacific Biosciences (2014). Template Preparation and Sequencing Guide. https://www.pacb.com/wp-content/uploads/2015/09/Guide-Pacific-Biosciences- Template-Preparation-and-Sequencing.pdf
Nguyen, J. (2021). Oxford Nanopore Sequencing. https://apollo-institute.org/oxford-nanopore-sequencing
Wang, Y., Zhao, Y., Bollas, A. et al. (2021). Nanopore sequencing technology, bioinformatics and applications. Nature Biotechnology, 39, 1348-1365. https://www.nature.com/articles/s41587-021-01108-x
Sanger sequencing vs NGS
AdvancedSeq (n.d.). About Sanger Sequencing. https://advancedseq.com/about-sanger-sequencing
Arteche-López, A. et al. (2021). Sanger sequencing is no longer always necessary based on a single-center validation of 1109 NGS variants in 825 clinical exomes. Sci Rep, 11, 5697.
https://doi.org/10.1038/s41598-021-85182-w
GenScript (2021). Sanger Sequencing vs. Next-Generation Sequencing (NGS). https://www.genscript.com/gene-news/sanger-sequencing-vs-next-generation- sequencing.html
Zhang, A. (2019). How does Next Generation Sequencing work? https://www.thetech.org/ask-a-geneticist/articles/2019/sanger-vs-next-gen- sequencing
Victoria Wang, X. et al. (2012). Estimation of sequencing error rates in short reads. BMC Bioinformatics, 13, 185.
https://doi.org/10.1186/1471-2105-13-185
Gorcenco, S. et al. (2020). New generation genetic testing entering the clinic. Parkinsonism & Related Disorders, 73, 72-84, https://doi.org/10.1016/j.parkreldis.2020.02.015
Sharma, R. (2022). NGS versus Sanger Sequencing for Clinical Decisions. https://www.clinicallab.com/trends/genomics/ngs-versus-sanger-sequencing-for- clinical-decisions-23214
Shaffer, C. (2019). Sanger Sequencing and Next-Generation Sequencing Compared. https://www.news-medical.net/life-sciences/Sanger-and-next-generation-compared. aspx
Birla, B. (2017). PacBio vs. Oxford Nanopore sequencing. https://blog.genohub.com/2017/06/16/pacbio-vs-oxford-nanopore-sequencing
Mobley, I. (2021). Long-read sequencing vs short-read sequencing. https://frontlinegenomics.com/long-read-sequencing-vs-short-read-sequencing
Illumina (n.d.). Read length recommendations. https://www.illumina.com/science/technology/next-generation-sequencing/plan- experiments/read-length.html
Cook, J. (2022). Illumina VS PacBio: What Sets these Companies Apart? https://bridgeinformatics.com/illumina-vs-pacbio-what-sets-these-companies-apart
Pacific Biosciences (n.d.). Revio System. https://www.pacb.com/revio
Grand View Research (n.d.). Short-read Sequencing Market Size, Share & Trends Analysis Report By Product (Instruments, Consumables), By Workflow, By Application, By End-Use, By Region, And Segment Forecasts, 2023 - 2030. https://www.grandviewresearch.com/industry-analysis/short-read-sequencing-market
Grand View Research (n.d.). Long Read Sequencing Market Size, Share & Trends Analysis Report By Product, By Technology (Nanopore Sequencing), By Workflow, By Application, By End-Use, By Region And Segment Forecasts, 2023 - 2030. https://www.grandviewresearch.com/industry-analysis/long-read-sequencing-market
Rhoads, A., Au, K. F. (2015). PacBio Sequencing and Its Applications. Genomics, Proteomics & Bioinformatics, 13(5), 278-289. https://www.sciencedirect.com/science/article/pii/S1672022915001345
Pacific Biosciences (2012). Detecting DNA Base Modifications Using Single Molecule, Real-Time Sequencing.
https://www.pacb.com/wp-content/uploads/2015/09/WP_Detecting_DNA_Base_ Modifications_Using_SMRT_Sequencing.pdf
Wenger, A. (2021). Methylation Detection with PacBio HiFi Sequencing. https://www.pacb.com/videos/methylation-detection-with-pacbio-hifi-sequencing
Illumina (n.d.). Illumina sequencing platforms. https://emea.illumina.com/systems/sequencing-platforms.html
Illumina (n.d.). Revealing the most challenging regions of the genome. https://emea.illumina.com/products/by-brand/complete-long-reads-portfolio.html
Pacific Biosciences (n.d.). Onso System. https://www.pacb.com/onso
Oxford Nanopore Technologies (n.d.). Sequencing short fragments with nanopore technology.
https://nanoporetech.com/applications/techniques/short-fragment-mode
Cairns, E. (2022). Pacbio is in it for the long run. https://www.evaluate.com/vantage/articles/interviews/pacbio-it-long-run
Pacific Biosciences (2022). PacBio Announces Onso, the Highly Accurate Short-Read Sequencing Platform.
https://www.pacb.com/press_releases/pacbio-announces-onso-the-highly-accurate- short-read-sequencing-platform
Oxford Nanopore Technologies (2021). Oxford Nanopore Tech Update: new Duplex method for Q30 nanopore single molecule reads, PromethION 2, and more. https://nanoporetech.com/about-us/news/oxford-nanopore-tech-update-new-duplex- method-q30-nanopore-single-molecule-reads-0
Udaondo, Z. (2021). Comparative Analysis of PacBio and Oxford Nanopore Sequencing Technologies for Transcriptomic Landscape Identification of Penaeus monodon. Life, 11(8), 862.
https://doi.org/10.3390/life11080862
Davison, A. (2018). World's longest DNA sequence decoded. https://www.bbc.com/news/science-environment-46046024
Oxford Nanopore Sequencing (n.d.). Portable sequencing using nanopore technology. https://nanoporetech.com/portable-sequencing
Enabling the Illumina DNA PCR-Free Library Prep kit on the MIRO CANVAS® NGS prep system
Kebschull JM et al. Nucleic Acids Res 2015; 43 (21): e143.
Yoo J et al. Poster 32 presented at the Association of Biomolecular Resources Facilities (ABRF) 2021 Virtual Annual Meeting; 7-11 March 2021.
Illumina DNA PCR-Free Prep. Available at:
https://www.illumina.com/products/by-type/sequencing-kits/library-prep-kits/dna-pcr- free-prep.html accessed April 2021.
Automating PacBio® SMRTbell® whole genome sequencing library prep on MIRO CANVAS®
Wenger AM, Peluso P, Rowell WJ, Chang P, Hall RJ, Concepcion GT, Ebler J, Fungtammasan A, Kolesnikov A, Olson ND, Töpfer A, Alonge M, Mahmoud M, Qian Y, Chin C, Phillippy AM, Schatz MC, Myers G, DePristo MA, Ruan J, Marschall T, Sedlazeck FJ, Zook JM, Li H, Koren S, Carroll A, Rank DR, Hunkapiller MW. Accurate circular consensus long-read sequencing improves variant detection and assembly
of a human genome. Nature Biotechnology. 2019 Oct;37(10):1155-1162. doi: 10.1038/ s41587-019-0217-9. Epub 2019 Aug 12. PMID: 31406327.
Logsdon GA, Vollger MR, Eichler EE. Long-read human genome sequencing and its applications. Nature reviews. Genetics. 2020 Oct;21(10):597-614. doi: 10.1038/ s41576-020-0236-x. Epub 2020 Jun 5. PMID: 32504078.
Automating the Oxford Nanopore Ligation Sequencing Kit on MIRO CANVAS®
Wick RR, Judd LM, Gorrie CL, Holt KE. Completing bacterial genome assemblies with multiplex MinION sequencing. Microbial genomics. 2017 Sep 14;3(10):e000132. doi: 10.1099/mgen.0.000132. eCollection 2017 Oct. PMID: 29177090.
Petersen LM, Martin IW, Moschetti WE, Kershaw CM, Tsongalis GJ. Third-generation sequencing in the clinical laboratory: exploring the advantages and challenges of nanopore sequencing. Journal of Clinical Microbiology. 2020 Jan; 58(1): e01315-19. doi: 10.1128/JCM.01315-19. PMID: 31619531.
Deamer D, Akeson M, Branton D. Three decades of nanopore sequencing. Nature Biotechnology. 2016 May 6; 34(5): 518-524. doi: 10.1038/nbt.3423. PMID: 27153285.
Laver T, Harrison J, O'Niell PA, Moore K, Farbos A, Paszkiewicz K, Studholme DJ. Assessing the performance of the Oxford Nanopore Technologies MinION. Biomolecular Detection and Quantification. 2015 Mar;3:1-8. doi: 10.1016/j. bdq.2015.02.001. PMID: 26753127.
Implementing the Twist Human Core Exome Kit on MIRO CANVAS®
Rabbani B et al. The promise of whole-exome sequencing in medical genetics. J Hum Genet 2014; 59: 5-15.
https://doi.org/10.1038/jhg.2013.114
Lelieveld SH et al. Comparison of exome and genome sequencing technologies for the complete capture of protein-coding regions. Hum Mutat 2015; 36: 815-822. https://doi.org/10.1002/humu.22813
Holland I and Davies JA. Automation in the life science research laboratory. Front Bioeng Biotechnol 2020; 8: 571777.
https://doi.org/10.3389/fbioe.2020.571777
Twist Bioscience. Human core exome kit. Available at: https://www.labline.it/wp-content/uploads/2020/01/ProductSheet_NGS_ ExomeKit_26Jun 19_Rev2.1.pdf. Accessed Feb 2022.
Miroculus. New Class of Technology. Available at: https://miroculus.com/technology/. Accessed February 2022.
Miroculus, Inc. Miro Universal Library Prep (Twist) Protocol. Available at: https://miroculus.com/. Accessed: Feb 2022.
Miroculus, Inc. Miro Human Exome Hyb Capture (Twist) Protocol. Available at: https://miroculus.com/. Accessed: Feb 2022.
4.4 Technician's best friend - solving the dog epigenome to advance oncology
1. Son KH, Aldonza MBD, Nam AR, et al. Integrative mapping of the dog epigenome: reference annotation for comparative inter-tissue and cross-species studies. bioRxiv. Published online July 22, 2022:2022.07.22.501075. doi:10.1101/2022.07.22.501075