Industry Expertise Improves Capsid Separation
Preliminary testing and collaboration can combat the many pitfalls of scaling up viral vector production
Viral vectors are used to deliver genetic material into cells for research and therapeutics. Work with viral vectors aids our understanding of genetics, molecular biology, and disease mechanisms by examining the effect of knocking specific genes and mutations in or out of a cell or organism. Viral vectors are used for generation of a broad range of therapeutics for leukemia, hemophilia, severe combined immunodeficiency, sickle cell disease, Covid-19 vaccines and others. For many of these applications, large-scale production of viral vectors is essential for sustainable solutions. Industry partners with expertise in effective and financially feasible methodologies can help labs avoid common pitfalls when scaling up viral vector production.
The complexity of viral vector production
Viral vector production is a multi-step process in which the success of each subsequent step depends heavily on the success of the previous, explains Åsa Hagner McWhirter, PhD, a principal scientist at Cytiva with expertise in downstream processing and analysis.1 Overall process recovery of high-quality product is the most important value, but the sum of each step together will result in the overall recovery rate. For example, in a five-step viral vector purification process where each step results in approximately 80 percent yield, the overall recovery rate for the full five-step process would be approximately 30 percent.
This dependency presents a major pitfall of viral vector production—step-specific problems that lead to overall reduced recovery. Optimizing each step is important and can increase the likelihood of higher recovery rates.
How to maximize the recovery rate of large-scale viral vector production: Tips from the bench
As McWhirter explains, different viral vectors come with their own challenges. For example, lentiviruses are enveloped viruses with an outer membranous layer that possess limited stability, being highly sensitive to environmental conditions including pH, temperature, shear forces, and salt concentrations. Workflows for these vectors need careful consideration at each step to minimize loss of functional activity due to handling and conditions, adjusting run times or removing steps as needed to improve vector stability. They may require dilution or specific stabilizers, adding complexity to workflows.
Adenoviruses and adeno-associated viruses (AAV) are non enveloped viruses with a much more stable protein capsid, McWhirter continues, but instead usually require whole cells to be lysed during harvest, which form a greater purification challenge. This is amplified by a tendency for viral particles to aggregate and adhere to cellular components. Producing good starting material for high-quality results relies on an optimized filtration procedure to remove the many cellular components present in lysates. In the same vein, ensuring there are no other materials of animal origin (such as serum) present during these processes is important from a regulatory perspective. Adenoviruses in particular are challenging to separate from host cell DNA. One challenge for AAV production is that it produces a large number of empty and partially full capsid product impurities, with only about 20 to 40 percent of capsids being full at harvest, creating yield and efficiency challenges during purification.
For labs that will be scaling up production, McWhirter recommends keeping a few key considerations in mind based on years of project experience and collaborations. It’s important that the methods used in early stages are compatible with large-scale production for good manufacturing practice (GMP) from the start or are easily adapted. Most methods developed for small-scale research settings simply cannot be feasibly translated to large-scale production, which is why leaning on industry experts with direct experience on large-scale production can reduce resources spent troubleshooting and optimizing protocols. Each factor of the multi-step process must be validated, fit for purpose (which can be confirmed and quantified with analytics), and robust enough for the scale-up process.
Similarly, labs planning for future scale-up should design processes early to maximize speed and productivity through cost-efficient and performance-efficient process steps. Pharmaceutical therapeutics must be affordable for widespread use. This means that not only must the process, reagents, and materials be cost-effective, but production and purification during viral vector process development also needs to be efficient and strive towards good recovery.
Small- and large-scale models are not always directly comparable
Choosing to run small-scale models prior to scaling up experimental procedures for producing and purifying viral vectors can provide answers and projections for future work. Some chromatography labs have taken to using mechanistic modeling for optimization and increased process understanding to determine how robust different steps are, and how performance is affected by different conditions and experimental setups, McWhirter explains. Another example of this is evaluating whether incorporating high throughput automated tools like multi-well plates, magnetic beads, or liquid handlers impact results.
Small-scale experimental results may be used to predict the outcome of large-scale procedures, but this must be confirmed before results can be relied on. Analytics may not perform well if the scale is too small because the amounts of impurity or target may be below the detection limit of the assays used. Small-scale set-ups may miss factors that are exclusively related to large-scale production. When performing process development for large-scale viral vector production, staff must test scalability, perform replicates, and plan for large-scale GMP setups to ensure the value of the work.
Traversing the waves of regulatory requirements
Regulatory and compliance requirements add another layer of complexity to viral vector work. Characterization of the final product, analysis, and detection of the exact constituents is required for any viral vector product intended for eventual therapeutic use. Batch-to-batch consistency of viral vector products is required and is only attainable by using a robust upstream and downstream process. For example, the number of AAV serotypes and engineered variants makes it extremely difficult to apply a single platform as well as characterize and maintain consistency between batches. This problem is frequently discussed at conferences, McWhirter states, and solutions are still being identified.
A case study in viral vector production innovation and problem-solving
McWhirter describes a recent example of Cytiva’s work that employed some of the above tactics to maximize full capsid yield for recombinant AAV (rAAV) vectors with an efficient purification process. Two key challenges for rAAV vector production are improving overall yield with an optimized affinity capture step and minimizing product impurities (empty capsids) with an effective polishing step that improves separation of empty and full capsids at scale.
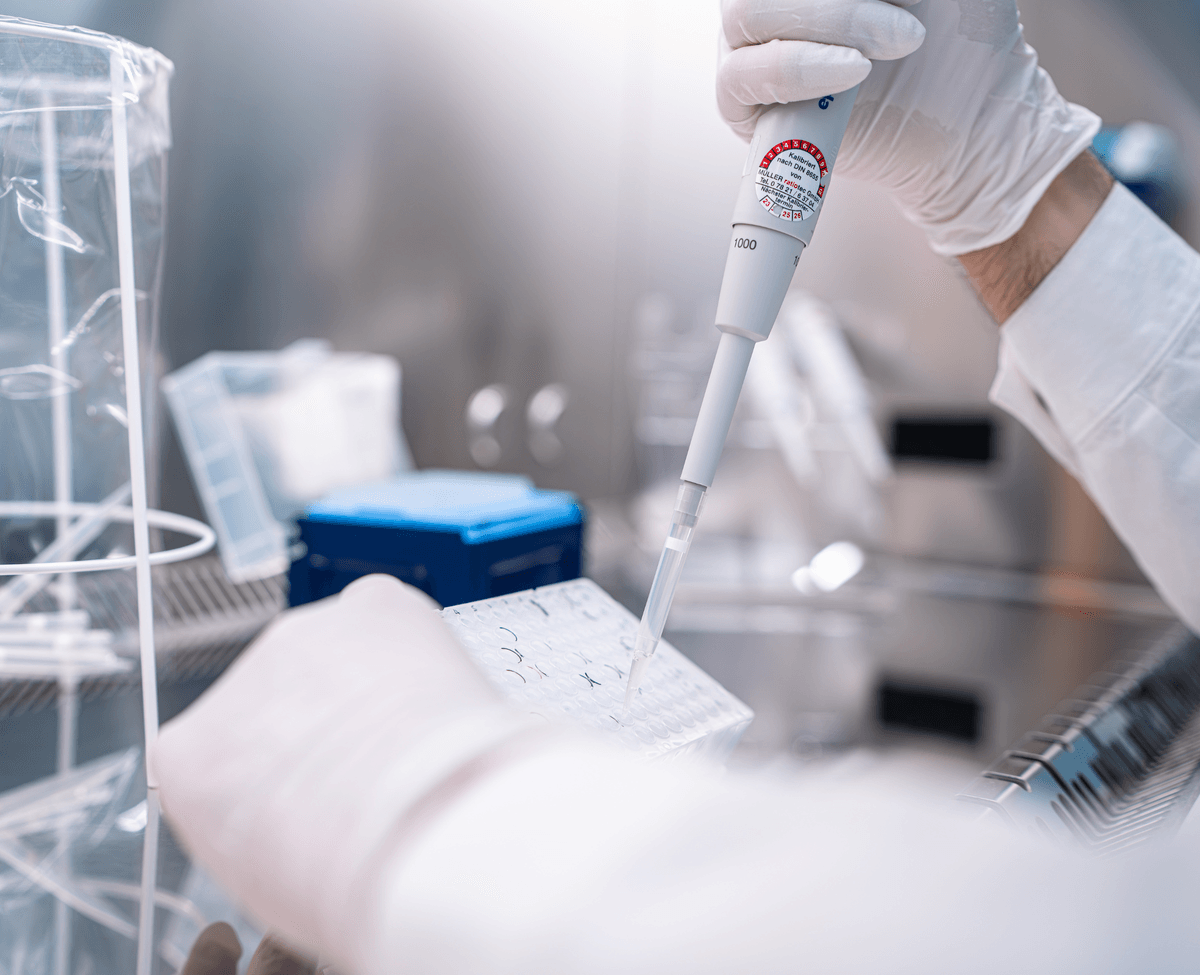
Cytiva recently published their protocol for optimizing capture and polishing steps suitable for large-scale rAAV purification. The method uses a specific affinity chromatography resin (CaptoTM AVB) to efficiently bind and concentrate rAAV with elution conditions optimized for rAAV2 and rAAV5 serotype to increase the viral particle recovery. For effective separation of full and empty capsids, the method involves a carefully selected anion exchange resin and optimized wash and elution protocols employing MgCl2, elution salt, and additives, using either gradient or isocratic elution. While the former elution protocol requires less pre-optimization, the latter gives better performance and is preferred for large-scale, simplified production steps. Overall, the method demonstrates an efficient, scalable alternative to small-scale ultracentrifugation to enrich full capsids.
The challenge of rAAV vector enrichment given the often-low percentage of full capsids in harvest material and diverse serotypes is one McWhirter’s team has worked on extensively for the last two to three years, with promising developments for a universal separation protocol.
They have found dextran surface extenders on anion exchange resins (CaptoTM Q) increases separation considerably. Differing isoelectric points for full and empty capsids (5.9 and 6.3 pI respectively) allow for differential elution in ion exchange chromatography, but the minute charge difference often translates to overlapping peaks using anion exchange. This creates a tradeoff between purity and yield. Dextran surface extenders with Q ligands are flexible for binding to large entities like viruses, with secondary structures that may change based on conductivity. These specialized resins demonstrate clearly enhanced separation for AAV vectors experimentally, particularly in combination with optimal magnesium chloride concentration and isocratic salt elution steps rather than linear salt gradients.
The team has developed a protocol using a single buffer with sodium acetate as an elution salt that works for all AAV serotypes tested to date (serotypes 2, 5, 6, 8, and 9) with good results. Prescreening for each capsid serotype and variant is needed to be able to determine the optimal conditions for a final 2-step protocol. Standard buffers are recommended, but some adjustments may be required depending on capsid. The protocol demonstrated more than 80 percent viral genome recovery with full-capsid purity over 75 percent and up to 95 percent by analytical ultracentrifugation (AUC). For some serotypes, a reduction of partial capsids was seen. Further, this method demonstrates performance on par with cesium chloride ultracentrifugation when using Capto Q for the commonly used AAV 9 serotype. Initially assessed using qPCR, ELISA, and UV260:280 ratio, the high purity was recently confirmed using AUC, which is the gold standard analysis of empty, partial and full capsids for this type of work. While further testing is still underway, according to McWhirter, this robust, scalable protocol has the potential to be universally applied with changes in buffer concentration only during wash steps.
The future of large-scale viral vector production
With each stage in viral vector production affecting the next, collaboration between labs and manufacturers would accelerate progress, building on lessons learned, says McWhirter.
While Cytiva’s new protocol is a positive step in the right direction for maximizing empty capsid removal, there is much work to be done in continuing to improve the separation between full and partially filled vector capsids containing a range of different truncated forms of viral genomes. Utilization of innovative products and solutions, reassessment of methods, and process intensification are the best ways to continue to adapt and change viral vector production and purification for the better. Only by looking holistically at upstream, downstream and analytics at each fundamental step of viral vector production can the overall quality and efficiency of therapeutic production be improved.
- Åsa Hagner McWhirter, PhD, Viral Vectors Downstream Bioprocessing R&D, Cytiva
Åsa has been with Cytiva based in Uppsala, Sweden since 2003 and is a downstream and analytics SME, with a broad and deep understanding of viral vector processing. Due to her long experience and from customer interactions she has gained insights into common challenges and pitfalls for processing and analytics of viral vectors and vaccines. She has been leading teams developing innovative new tools to improve performance and productivity of viral vector processing. Åsa holds a PhD in Medical Biochemistry from Uppsala University in 1999 based on research around biosynthesis of proteoglycans.