Advances in Biomaterials: Engineering the Future of Healthcare
Innovations in biomaterials enable better medical treatments and personalized healthcare solutions
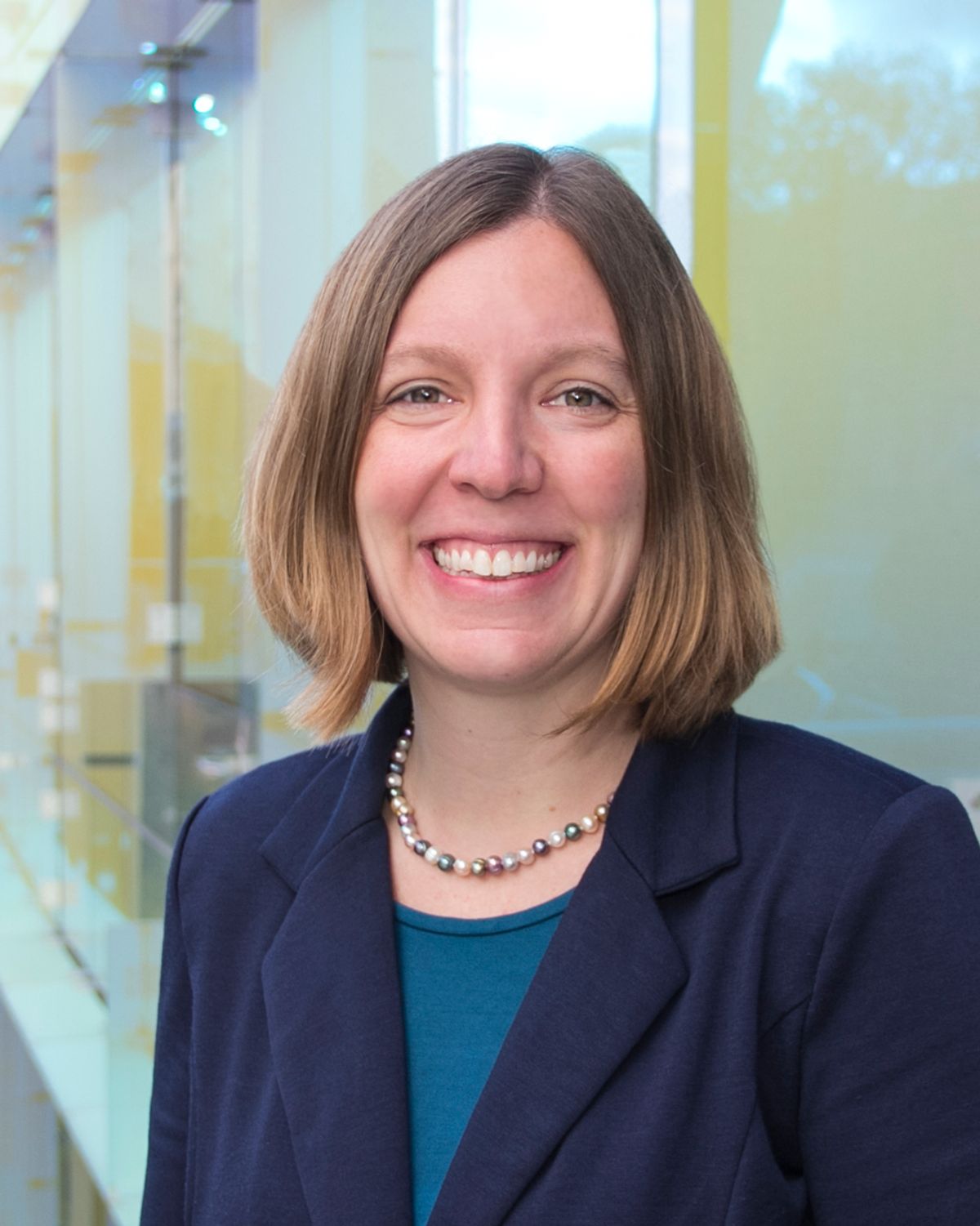
Biomaterials with unique properties have emerged with the potential for advances in areas such as heart valve prostheses, precise drug delivery systems, and tissue engineering. Rosalyn Abbott, PhD, assistant professor in biomedical engineering and material science engineering at Carnegie Mellon University, discusses the current landscape of biomaterials research and how it is opening new horizons for personalized and more effective healthcare solutions.
Q: What sparked your interest in biomaterials?
A: My interest in biomaterial research stemmed from its key role in tissue engineering. One of the main goals of tissue engineering is to build structures that can repair or replace damaged tissues or organs in patients. In the body, tissues are assembled of cells which are surrounded by an extracellular matrix, a large network of proteins and other molecules that provides the scaffolding and cues for cells to function. Biomaterials are used to mimic this extracellular matrix, providing structure for the cells to grow in 3D. In my lab at Carnegie Mellon University (CMU), we focus on fat tissue engineering and use different biomaterials for diverse tissue engineering applications including regenerative medicine, disease modeling, and even the creation of meat products for human consumption.
Q: Can you explain what biomaterials are and give some examples of their applications in healthcare?
A: Biomaterials are defined as any material that interfaces with biological systems. Biomaterials can be used to rebuild, enhance, or replace any tissue or function in the body. There are many examples of biomaterials that are used regularly in healthcare for a variety of applications. Some common examples are heart valve prostheses, total hip replacements, dental implants, and intraocular lenses. Biomaterials can be metals, ceramics, or polymers. They can be made synthetically or derived from natural sources. For example, my lab often grows cells in polymeric silk scaffolds where the material was extracted from silkworm cocoons.
Q: What are the most recent advances in biomaterials, and how do you see these advances impacting society in the coming years?
A: The field initially started with the goal of biomaterials causing a minimal negative response (like inflammation) when implanted. Today, biomaterials have evolved from passive supports to actively initiating specific responses in the body. They can be designed to promote regenerative responses, deliver drugs and vaccines, or improve wound healing. They can be designed to enhance cell attachment (for tissue engineering approaches) or block cell or protein attachment (in the case of a stent or heart valve where cellular attachment would block blood flow). Biomaterials can even be 3D printed into anatomically correct shapes to fit patient-specific defects. Collectively, these advances will lead to better integration of biomedical interventions with the human body.
Q: Can you discuss any research project you're currently involved in or planning? What kind of future impacts do you anticipate from these projects?
A: My lab is using non-invasive methods, like ultrasound, to control how silk scaffolds break down—or degrade—over time. This approach allows us to tailor biomaterial degradation to a patient’s unique regenerative environment. In a recent publication in Advanced Healthcare Materials, we show for the first time the capability to finely tune silk degradation on demand. Controlling tissue biomaterial interactions non-invasively, in real time, based on how the patient is healing will minimize maladaptive immune responses and scarring. In the future, we expect it will be possible to tailor biomaterial breakdown to the unique healing environments of different patients; for example, triggering degradation to occur faster in younger patients who generally heal faster, and allowing the biomaterial to slowly degrade in older patients that do not heal as quickly.
Q: Could you share an example of a biomaterial innovation recently moving from research to practical application? What problem does it solve, and how?
A: As I mentioned previously, biomaterials are now being developed to actively elicit specific responses in the body. A recent biomaterial innovation that moved from research to widespread adoption in a public health emergency was the mRNA vaccines. The mRNA vaccines for the COVID-19 pandemic used sophisticated biomaterial carriers like lipid nanoparticles with polymers, peptides, and a cationic nano-emulsion. These biomaterials were designed to safely deliver the mRNA cargo and boost immunity to viral infections. Importantly, this new technology allowed the vaccine to be developed rapidly, while traditional vaccine development usually takes several years. Improving immunity to COVID-19 is just the tip of the iceberg for these biomaterials. In the future, these biomaterial carriers will deliver different mRNA cargo to defend against other diseases. This technology will allow vaccines to be quickly produced and distributed on a large scale within weeks of identifying a specific agent that causes a disease.
Q: How is adopting AI and advanced bioinformatics accelerating innovation in biomaterials?
A: Both techniques can use computation to rapidly iterate on different design parameters that experimentally would take too many resources and time. These tools can be used to minimize experiments that need to be performed to optimize biomaterial formulations, modify existing biomaterials to improve performance, optimize 3D printing parameters, simulate immune system responses to biomaterials, and tailor biomaterial degradation to match the new growth of tissue into the biomaterial.
Q: What are the biggest challenges or limitations facing the development of new biomaterials, and how is the field working to overcome them?
A: The current “menu” of biomaterials that are used in the body is relatively small given the diverse applications of biomaterials in the body. Part of the reason why the choices are limited revolves around the body being a harsh environment for implanting materials. For example, approximately two-thirds of our body is water, which causes metals to corrode. Cells also actively try to break down foreign objects by releasing chemical species. The mechanical environment in the body further drives biomaterial selection, as high forces can wear down implants. Finally, once a biomaterial is developed, it must be approved by the FDA for a specific application, which requires extensive, specific, and time-consuming studies to ensure the material's safety and effectiveness for its intended use. Despite these constraints, the biomaterial field has made considerable progress. For example, surface coatings can minimize corrosion. Fundamental research has also focused on material factors that regulate cell behaviors and modulate immune responses. Modification of existing material strategies can also be used to speed up FDA approval. This is an exciting time for biomaterial research as fundamental understandings of biomaterial interactions on the body can drive innovation to develop better biomaterials for diverse applications.
Rosalyn Abbott is an assistant professor in biomedical engineering and material science engineering (courtesy) at Carnegie Mellon University. Her lab focuses on fat tissue engineering and examining the role of lipid accumulation in a variety of diseases and applications. This general approach is applied to (1) engineer fat grafts to increase retention and functionality of reconstructive surgery, (2) develop disease models, (3) increase the number of donor livers available for transplantation via defatting protocols, and (4) cultivate high lipid content meat products to decrease the environmental impact of traditional agriculture methods.